Difference between revisions of "Pediococcus"
(standardizing headings) |
|||
(278 intermediate revisions by 4 users not shown) | |||
Line 1: | Line 1: | ||
− | + | [[File:Pedio.jpg|thumb|200px|right|[https://www.instagram.com/wildandsour/ Pediococcus - picture taken by Per Karlsson]]] | |
− | ''Pediococcus'' | + | '''''Pediococcus''''' (often referred to by brewers as "Pedio") are Gram-positive lactic acid bacteria (LAB) used in the production of Belgian style beers where additional acidity is desirable. They are native to plant material and fruits <ref name="ucdavis">[http://wineserver.ucdavis.edu/industry/enology/winemicro/winebacteria/pediococcus_damnosus.html Viticulture & Enology. UC Davis website. Pedioccous damnosus. Retrieved 07/28/2015.]</ref>, and often found in [[Spontaneous_Fermentation|spontaneously fermented]] beer as the primary source of lactic acid production (with ''P. damnosus'' being the only species identified in [[Lambic]]) <ref>[http://journals.plos.org/plosone/article?id=10.1371/journal.pone.0095384 The Microbial Diversity of Traditional Spontaneously Fermented Lambic Beer. Freek Spitaels, Anneleen D. Wieme, Maarten Janssens, Maarten Aerts, Heide-Marie Daniel, Anita Van Landschoot, Luc De Vuyst, Peter Vandamme. April 18, 2014.]</ref><ref>[[Scientific_Publications#Lambic_and_Spontaneous_Fermentation|Multiple Scientific publications linked on MTF.]]</ref>. It is also seen as a major source of beer contamination in commercial breweries due to its ability to adapt to and survive in beer. The ability to grow in beer is strain dependent rather than species dependent, however, genetic differences indicate that ''P. damnosus'' and ''P. claussenii'' are better adapted to surviving in beer than ''P. pentosaceus'' <ref name="Snauwaert">[http://www.biomedcentral.com/content/pdf/s12864-015-1438-z.pdf Comparative genome analysis of Pediococcus damnosus LMG 28219, a strain well-adapted to the beer environment. Isabel Snauwaert, Pieter Stragier, Luc De Vuyst and Peter Vandamme. 2015.]</ref>. Like many bacteria, pediococci have the ability to [https://en.wikipedia.org/wiki/Horizontal_gene_transfer transfer genes horizontally] without reproduction <ref name="Snauwaert"></ref>. They are generally considered to be facultative anaerobes, which means they grow anaerobically but can also grow in the presence of oxygen <ref>[http://textbookofbacteriology.net/lactics.html Lactic Acid Bacteria. Todar's Online Texbook of Bacteriology. Kenneth Todar, PhD. Pg 1. Retrieved 08/09/2015.]</ref>. Some species/strains (including individual strains of ''P. damnosus'') can have their growth and acid production inhibited by oxygen <ref name="NAKAGAWA">[https://www.jstage.jst.go.jp/article/jgam1955/5/3/5_3_95/_article TAXONOMIC STUDIES ON THE GENUS PEDIOCOCCUS. ATSUSHI NAKAGAWA, KAKUO KITAHARA. 1959.]</ref>, while some will have better growth and produce more acid in the presence of oxygen (microaerophilic) <ref>[http://www.ncbi.nlm.nih.gov/pmc/articles/PMC357257/ THE NUTRITION AND PHYSIOLOGY OF THE GENUS PEDIOCOCCUS. Erling M. Jensen and Harry W. Seeley. 1954.]</ref><ref>[http://www.microbialcellfactories.com/content/8/1/3#B5 Pediocins: The bacteriocins of pediococci. Sources, production, properties and applications. Maria Papagianni and Sofia Anastasiadou. 2009.]</ref>. Strains found in beer are hop tolerant <ref>[http://www.biomedcentral.com/1471-2164/16/267 Comparative genome analysis of Pediococcus damnosus LMG 28219, a strain well-adapted to the beer environment. Isabel Snauwaert, Pieter Stragier, Luc De Vuyst and Peter Vandamme. April 2015.]</ref>. Due to their continued metabolism of longer chain polysaccharides, acid production will increase with storage time. ''Pediococcus'' can form a [[pellicle]]. |
− | See [[Lactobacillus]], [[Brettanomyces]], [[Saccharomyces]], | + | ''Pediococcus'' may also cause “ropiness” (also called a "sick beer") due to the production of exopolysaccharides when exposed to a fresh sugar source. "Ropy" or "sick" beer is more viscous and, in extreme circumstances, can form strands. Sickness effects mostly the mouthfeel and appearance of the beer, and may have no influence on the flavor. It is considered a temporary flaw in sour beer. Some brewers, including Vinnie Cilurzo from Russian River Brewing and some Belgian lambic producers, claim that after the ropiness goes away (generally in 3-6 months <ref name="ropy_time"></ref>) it produces a deeper acidity and mouthfeel, and is viewed as a positive process in the production of sour beer <ref>[http://www.xxlbrewing.com/hb/sour_beer/img_09.html Cilurzo, Vinnie. AHA Sour Beer presentation. 2007.]</ref>. For other brewers, ropy beer is seen as a nuisance due to the beer needing to be aged for a longer period of time, especially when it occurs shortly after bottling. ''Pediococcus'' species can also produce diacetyl with extended storage time <ref name="Garcia-Garcia" />. ''[[Brettanomyces]]'' can break down exopolysaccharides and diacetyl produced by ''Pediococcus'' and the two are often used together. |
+ | |||
+ | See ''[[Lactobacillus]]'', ''[[Brettanomyces]]'', ''[[Saccharomyces]]'', [[Mixed Cultures]], [[Kveik#Commercial_Availability|Kveik]], and [[Nonconventional Yeasts and Bacteria]] charts for other commercially available cultures. | ||
+ | |||
+ | ==Introduction of Characteristics and Taxonomy== | ||
+ | ''Pediococcus'' can either improve or detract from fermented food products, including beer, wine, and cider. They play an important role in the fermentation of some meats and cheeses. They tend to be the primary souring microorganism in the production of [[Lambic|Belgian lambic]]. Some species have also been isolated from plants, fruit, and fermenting plant material. In wine, ''P. parvulus'' is the most common species found, and in beer ''P. damnosus'' is the most common. ''P. pentosaceus'' tends to be more hop sensitive than ''P. damnosus'' <ref name="Wade_2018">[https://onlinelibrary.wiley.com/doi/full/10.1111/ajgw.12366 Role of Pediococcus in winemaking. M.E. Wade, M.T. Strickland, J.P. Osborn, C.G. Edwards. 2018. DOI: https://doi.org/10.1111/ajgw.12366.]</ref>. | ||
+ | |||
+ | Currently, there are 11 recognized species of ''Pediococcus''. They are ''P. acidilactici'', ''P. argentinicus'', ''P. cellicola'', ''P. claussenii'', ''P. damnosus'', ''P. ethanolidurans'', ''P. inopinatus'', ''P. parvulus'', ''P. pentosaceus'' (subspecies ''pentosaceus'' and ''intermedius''), ''P. siamensis'', and ''P. stilesii''. ''P. cerevisiae'' was reclassified into either ''P. damnosus'' or ''P. pentosaceus''. Other species of ''Pediococcus'' have also been reclassified to other genera in the last couple of decades. ''P. dextrinicus'' is now classified as ''Lactobacillus dextrinicus'', ''P. urinae-equi'' is now classified as ''Aerococcus urinae‐equi'', and ''P. halophilis'' is now classified as ''Tetragenococcus halophilis'' <ref name="Wade_2018" />. | ||
+ | |||
+ | Pediococci are described as coccoidal (spherical) or ovoid (egg-shaped) in shape. They are Gram-positive, non-motile (not capable of moving on their own), and non-spore forming. They are obligate homofermentive and typically do not produce CO<sub>2</sub>, ethanol, or acetic acid, although there are a few exceptions to this in the literature. They do not produce [https://en.wikipedia.org/wiki/Catalase catalase] (except for some ''P. pentocaseus'' strains which were reported to have pseudo-catalase activity by Simpson and Taguchi 1995) or [https://en.wikipedia.org/wiki/Oxidase oxidase] enzymes. Because of the way that ''Pedioccous'' cells divide, they often appear stuck together in pairs or clumps. They are the only lactic acid bacteria found in wine and beer to do this, so they are easily identifiable at the genus level under a microscope based on their tendency to clump together. When grown on agar that is supplemented with 100 mg/L of pimaricin, the colonies are white-grey with a diameter of about 1 mm. Ropy strains have a high elasticity, and when touched with a needle, long threads can be drawn and sometimes the colonies completely stick to the needle. See [https://onlinelibrary.wiley.com/doi/full/10.1111/ajgw.12366 table 2 from Wade et al. (2018)] for more species identification indicators and what carbohydrates different species can ferment <ref name="Wade_2018" /><ref name="Oevelen_1979">[https://www.tandfonline.com/doi/abs/10.1094/ASBCJ-37-0034 D. Van Oevelen & H. Verachtert (1979) Slime Production by Brewery Strains of Pediococcus Cerevisiae, Journal of the American Society of Brewing Chemists, 37:1, 34-37, DOI: 10.1094/ASBCJ-37-0034.]</ref>. | ||
+ | |||
+ | See also: | ||
+ | * [https://wol-prod-cdn.literatumonline.com/cms/attachment/fcb6edb6-00be-4757-822c-be0a341a4191/ajgw12366-fig-0001-m.jpg Microscopy image of ''Pediococcus''.] | ||
==Commercial Pediococcus Cultures== | ==Commercial Pediococcus Cultures== | ||
Line 10: | Line 22: | ||
! Name !! Mfg# !! Taxonomy !! Note | ! Name !! Mfg# !! Taxonomy !! Note | ||
|- | |- | ||
− | | [[ | + | | [[Bootleg Biology]]/[[Spot Yeast]] || Sour Weapon P (''Pediococcus pentosaceus'' Blend) || ''P. pentosaceus'' blend || Perfect for acidifying unhopped wort quickly for kettle or “quick” sours. At 98F, it’s capable of achieving a pH of 3.3 within 18 hours. At 84F, it can reach a pH of 3.5 within 24 hours. With more time, a terminal pH of 3.1 may be reached. ''P. pentosaceus'' can also be used for long-term sours. It is capable of growing and producing lactic acid in worts with IBUs as high as 30, though it is recommended for unhopped worts as IBUs over 10 may prevent significant quick souring. At ~30 IBU, souring occurs in 2-3 months <ref>[https://www.facebook.com/groups/MilkTheFunk/permalink/1302981419730069/?comment_id=1870460696315469&comment_tracking=%7B%22tn%22%3A%22R0%22%7D Justin Amaral and Per Karlsson on Bootleg biology Sour Weapon hop tolerance. milk The Funk Facebook group. 11/2/2017.]</ref>. This culture may produce antimicrobials called bacteriocins or pediocins. These can inhibit and kill similar species of bacteria like Lactobacillus and other Pediococcus species in mixed-culture fermentations. Read Bootleg Biology's [https://www.facebook.com/BootlegBiology/photos/a.148869931970401.1073741829.124634287727299/465185997005458/?type=1&theater Facebook post] regarding bacteriocins for more info. No signs of ropiness (exopolysaccharides) have occurred in testing <ref>[http://bootlegbiology.com/product/sour-weapon-pediococcus-pentosaceus-blend/ Bootleg Biology website. Retrieved 05/06/2016.]</ref>. It is still unknown how hops will affect souring in a long term scenario. Bootleg Biology is still researching long term effects and awaiting peoples feedback as of 5/23/2016. |
+ | |- | ||
+ | | [[East Coast Yeast]] || ECY33 || ''P. parvulus'' || Isolated from lambic which was refermented with grapes, this strain of ''Pediococcus'' produces lactic acid, diacetyl, and may cause ropiness in beer. Always add ''Brettanomyces'' where ''Pediococcus'' is used <ref name="ecy_website">[http://www.eastcoastyeast.com/wild-stuff.html "Wild Yeast / Brettanomyces / Lactic Bacteria". East Coast Yeast website. Retrieved 04/27/2018.]</ref>. | ||
+ | |- | ||
+ | | [[Escarpment Laboratories]] || Pediococcus Blend || Two ''Pediococcus'' strains (''P. damnosus'' and ''P. pentosaceus'') || This blend of 2 hop-resistant Pediococcus strains (P. damnosus and P. pentosaceus) is intended for use in long-term souring. Neither strain has been observed to produce exopolysaccharides (EPS). We recommend 15 IBU or less in the first generation. | ||
+ | |- | ||
+ | | [[Inland Island Brewing & Consulting|Inland Island Yeast Laboratories]] || INISBC-998 || ''P. damnosus'' || Gram positive cocci that produces lactic acid. Also produces diacetyl and several proteins that may cause a "rope" to form in the beer. Rope will disappear with time. Oxygen and hop sensitive. 75-90°F Temperature Range. '''No longer available.''' | ||
|- | |- | ||
− | | [[Wyeast]] || 5733 || damnosus || May cause “ropiness” and produce low levels of diacetyl with extended storage time. Temp range: 60-95° F (15-35° C). | + | | [[Mainiacal Yeast]] (CLOSED) || MYPP1 || ''P. pentocaceus'' || Isolated from a growing marijuana plant. No production of diacetyl or EPS, with a clean acidity. Ferment at 70-100°F <ref name="Amaral_Mainiacal">Private correspondence with Justin Amaral by Dan Pixley. 01/24/2018.</ref>. Commercial pitches only. |
+ | |- | ||
+ | | [[Mainiacal Yeast]] (CLOSED) || MYPD1 || ''P. damnosus'' || Isolated from a bottle of belgian lambic. Produces diacetyl and EPS, so use in conjunction with ''Brettanomyces''. Ferment at 70-90°F <ref name="Amaral_Mainiacal" />. Commercial pitches only. | ||
+ | |- | ||
+ | | [[Omega Yeast Labs]] || OYL-606 || ''P. damnosus'' || This modestly hop tolerant Pedio strain produces a clean lactic tang over time. The strain can produce diacetyl so it is often paired with one or more Brett strains (to consume the diacetyl). While more hop tolerant than the Lacto Blend (OYL-605), IBUs over 5-10 IBU may inhibit souring. Souring time can vary depending on IBU level <ref>[https://omegayeast.com/yeast/bacterial-cultures/pediococcus Pediococcus OYL-606. Omega Yeast Labs website. Retrieved 06/11/2019.]</ref>. EPS production is unknown and has not been observed or reported <ref>[https://www.facebook.com/groups/MilkTheFunk/permalink/2719576444737219/?comment_id=2719963548031842&comment_tracking=%7B%22tn%22%3A%22R%22%7D Adi Hastings. Milk The Funk Facebook group on OYL Pediococcus EPS production. 06/11/2019.]</ref>. Commercial pitches only. | ||
+ | |- | ||
+ | | [[Propagate Lab]] || MIP-920 || ''P. damnosus'' || Will sour a finished beer over time to a pH of 3.5 - 3.1. It works well at room temperature and is hop tolerant. It may produce ropiness <ref>[http://www.propagatelab.com/mip-920pediodamn Propagate Labs website. MIP-920. Retrieved 06/20/2020.]</ref>. | ||
+ | |- | ||
+ | | [[RVA Yeast Labs]] || RVA 601 || ''P. damnosus'' || Lactic acid bacteria used in souring Belgian-style beers such as gueze and Lambic. Acid production increases with storage. Temperature range is 60-95°F. | ||
+ | |- | ||
+ | | [[White Labs]] || WLP661 || ''P. damnosus'' || High diacetyl (a.k.a. butter) producer and slow growing. Fermentation temp: 70-85°F (21-29.4° C). Attenuation: 65%. Cell count: 50-80 million cells/mL <ref name="WL_cellcounts">Private correspondence with White Labs Customer Service and Dan Pixley. 10/29/2015.</ref>. Homebrew vials contain 35 mL of slurry, and ~1.75-2.8 billion cells per vial <ref name="priess_pitches">[https://www.facebook.com/groups/MilkTheFunk/permalink/1170980479596831/?comment_id=1171066879588191&reply_comment_id=1171595552868657&total_comments=6&comment_tracking=%7B%22tn%22%3A%22R9%22%7D Conversation with Richard Priess regarding WL and Wyeast homebrew pitches. 10/30/2015.]</ref>. | ||
+ | |- | ||
+ | | [[Wyeast]] || 5733 || ''P. damnosus'' (previously listed as ''P. cerevisiae'') || May cause “ropiness” and produce low levels of diacetyl with extended storage time. Temp range: 60-95° F (15-35° C). Cell count: 1.0 x 10<sup>8</sup> (100 million) cells/mL <ref>[https://drive.google.com/folderview?id=0B8CshC9nxYHdZmE4MmoyLXA2WVk&usp=sharing Wyeast Specifications 2015 Retail Products. 2015.]</ref>. Homebrew packs contain 100-125 mL of slurry, and ~10-12.5 billion cells <ref name="priess_pitches"></ref>. | ||
|- | |- | ||
− | |||
|} | |} | ||
− | ==Manufacturer Tips== | + | ===Manufacturer Tips=== |
− | ===[[Wyeast]] on 5733=== | + | ====[[Bootleg Biology]] on Sour Weapon P==== |
− | + | This product, which is a blend of ''Pediococcus pentosaceus'', can be used for a kettle souring process or a mixed fermentation process. Jeff reported good growth results using 2 grams of calcium carbonate (chalk; CaCO3) per liter of wort for starters of Sour Weapon <ref name="mello_starter">[https://www.facebook.com/groups/MilkTheFunk/permalink/1369904163037794/?comment_id=1370329352995275&reply_comment_id=1370751796286364&comment_tracking=%7B%22tn%22%3A%22R5%22%7D Conversation on MTF with Jeff Mello regarding starters for Pediococcus. 08/08/2016.]</ref>. This same formula might benefit other ''Pediococcus'' starters as well. The CaCO3 serves as a buffering agent that keeps the starter pH from getting too low too fast, and is similar to the concept of buffered growth media for ''Lactobacillus'' (see [[Lactobacillus#Samuel_Aeschlimann.27s_Starter_Procedures|''Lactobacillus'' Starter]]). | |
+ | |||
+ | Regarding exposure to oxygen in general: | ||
+ | <blockquote> | ||
+ | "We don't propagate Sour Weapon on plates or at the liquid stage anaerobically, and definitely don't have problems with growth or souring. We have not tested pitching the culture in aerated wort, but there's certainly the chance that you could be increasing the likelihood of off flavors. Sour Weapon does still work very well when co-pitched with yeast in wort that has been aerated though. Hope that helps." - Jeff Mello of [[Bootleg Biology]] <ref>[https://www.facebook.com/groups/MilkTheFunk/permalink/1418878731473670/?comment_id=1419061968122013&comment_tracking=%7B%22tn%22%3A%22R%22%7D Discussion with Jeff Mello on MTF regarding Sour Weapon and oxygen. 09/27/2016.]</ref>. | ||
+ | </blockquote> | ||
+ | |||
+ | ====[[Wyeast]] on 5733==== | ||
+ | <blockquote> | ||
+ | "If using 1 pack of 5733 per 5 gallon batch; and either adding to secondary after alcoholic fermentation is complete, or co-inoculating with a Saccharomyces' strain, then a starter would not be necessary. If you did want or need to propagate, I'd recommend 2 liters of 1.030-1.040 wort per pack, incubated at 80-90*F, without agitation." - Michael Dawson, Wyeast. | ||
− | + | "For propagation, we recommend using 1.040 OG wort and incubating at 30-35*C without aeration for 48-96 hours; pH drop will indicate when it's ready to pitch. For co-inoculation or post-primary addition, we recommend 0.5 million cells per mL, which is the equivalent of 1 pack in 5 gallons/20 liters. For larger volumes, you can propagate and inoculate with the starter culture at a rate 2.5-5% of the total volume of the main batch." - Michael Dawson, Wyeast. | |
+ | </blockquote> | ||
− | ===[[White Labs]] on WLP661=== | + | ====[[White Labs]] on WLP661==== |
+ | <blockquote> | ||
"That one does well in 70-85 deg F. You can do a starter, but you shouldn't have to if you are doing a 5 gallon batch. It does take a while to sour, so just be patient and let it do it's thing." Sarah Neel, Sales and Customer Service, White Labs | "That one does well in 70-85 deg F. You can do a starter, but you shouldn't have to if you are doing a 5 gallon batch. It does take a while to sour, so just be patient and let it do it's thing." Sarah Neel, Sales and Customer Service, White Labs | ||
+ | </blockquote> | ||
+ | |||
+ | ===Tips From MTF=== | ||
+ | ====The Rare Barrel==== | ||
+ | <blockquote> | ||
+ | "We just performed an interesting experiment at The Rare Barrel with pedio. Jay, our head brewer and blender, wanted a more acidic beer that we could use as a blending component while also growing up our diminishing pedio culture. So we racked 2 oak barrels of gold fermented with [[Brettanomyces|Brettanomyces claussenii]] and White Labs ''Pediococcus damnosus'' (WLP661) into one of our 30 bbl batches of 12*p gold wort that had been acidified to about 4.5 pH in the kettle using lactic acid (our first hot side experiment!). No oxygen, really wanted to encourage the bacteria. Within 10 days the pH was 3.6 and the gravity 10.7. We were all surprised how quickly the pedio was working. We eventually racked a "splash" of fermenting gold with BSI Brett D and BSI Lacto D to drop the gravity. The beer had a bright acidity quickly and I was surprised at how well rounded the flavors were when we racked into barrels after a month in the fermenting vessel. | ||
− | + | This might be a little harder to do at home, but I think there's potential for interesting results. ''Pediococcus'' shines long term traditionally so I agree with the posts above, if you're going for quick acidity I'd go lacto, but I plan on playing around with early ''Pediococcus'' fermentations at home." - Mike Makris from The Rare Barrel <ref>[https://www.facebook.com/groups/MilkTheFunk/permalink/1041019779259569/?comment_id=1041073789254168&offset=0&total_comments=8 Conversation with Mike Makris on Milk The Funk.]</ref>. | |
− | + | </blockquote> | |
− | '' | ||
− | '' | + | ''Pediococcus'' has a reputation for being a "slow worker", meaning that it produces acidity overtime. Staff at The Rare Barrel noticed that pitching on second generations of White Labs WLP661 ''Pediococcus'' might help the ''Pediococcus'' produce acidity very quickly <ref name="SourHour29">[http://www.thebrewingnetwork.com/the-sour-hour-episode-29/ The Sour Hour Episode 29 on The Brewing Network, interview with The Rare Barrel staff. 02/19/2016.]</ref> (~19 minutes in). They speculate that oxygen exposure might inhibit acid production with the White Labs WLP661 ''P. damnosus'' strain, however this is purely speculative and anecdotal <ref name="SourHour29"></ref> (~32 minutes in). |
==Metabolism== | ==Metabolism== | ||
===Growth and Environment=== | ===Growth and Environment=== | ||
− | One study showed that | + | ''Pediococcus'' generally has a high tolerance to ethanol compared to other bacteria, and can range from 10-25% ABV, depending on the species and strain. ''P. damnosus'' is sensitive to warm temperatures. It is unable to grow at 35°C or higher. The optimal growth occurs at 22°C. Other species can be more tolerant of higher temperatures, for example, ''P. parvulus'' and ''P. inopinatus'' have been shown to grow between 12 to 40°C, with rapid death occuring at 45°C <ref name="Wade_2018" />. ''P. damnosus'' is sensitive to environments that contain NaCl, and will not grow with concentrations of 4% NaCl <ref name="ucdavis"></ref>. Most strains of ''P. claussenii'' can grow in beer. About half of the strains tested of ''P. damnosus'' can grow in beer, and none to very few strains of ''P. acidilactici'', ''P. parvulus'', and ''P. pentosaceus'' have been found to grow in finished beer. |
+ | |||
+ | Another environmental factor that can affect the growth and survival of ''Pediococcus'' is pH. ''P. damnosus'' is unable to grow at a pH of 8 or higher. One study showed that optimal growth for ''P. damnosus'' was observed in [http://www.neogen.com/Acumedia/pdf/ProdInfo/7406_PI.pdf MRS media] after ~84 hours with an initial pH of 6.7, and a final pH of 4.14, which occurred naturally from fermentation. The addition of bacteriological peptone, MnSO4, and Tween 80 also increased activity. Maximum cell densities of ''P. damnosus'' are around 4.3 billion cells/mL in MRS media starting at a pH of 5.5 <ref>[https://www.facebook.com/groups/MilkTheFunk/permalink/1347683325259878/?comment_id=1349386438422900&reply_comment_id=1350340544994156&comment_tracking=%7B%22tn%22%3A%22R%22%7D Conversation with Richard Preiss on MTF regarding Pediococcus cell density. 07/19/2016.]</ref><ref name="Nel">[http://www.ncbi.nlm.nih.gov/pubmed/11851822 Growth optimization of Pediococcus damnosus NCFB 1832 and the influence of pH and nutrients on the production of pediocin PD-1. Nel HA, Bauer R, Vandamme EJ, Dicks LM. 2001.]</ref>, but this is only in optimal conditions. Maximum cell density varies based on the conditions of the propagation with pH and nutrient demands being two of the main limiting factors <ref>[https://www.reddit.com/r/Homebrewing/comments/3qp7b7/advanced_brewers_round_table_neva_parker_white/cwh7iqq Neva Parker, Reddit thread. 10/29/2015.]</ref>. | ||
+ | |||
+ | Although more experiments are probably needed, agitation is believed to be an important factor for any species of microbe (yeast and bacteria). Gentle stirring on a stir plate or orbital shaker, or frequent gentle manual agitation leads to faster growth and a higher number of organisms. Agitation keeps the microbes in solution. It also maximizes the microbes' access to nutrients and disperses waste evenly. In a non-agitated starter, the microbes are limited to the diffusion rate of nutrients, leading to a slower and more stressful growth <ref>[https://www.facebook.com/groups/MilkTheFunk/permalink/1168024059892473/?comment_id=1174865305875015&reply_comment_id=1176092372418975&total_comments=1&comment_tracking=%7B%22tn%22%3A%22R9%22%7D Conversation with Bryan of Sui Generis Blog about starters and agitation. 11/09/2015.]</ref>. Although ''Pediococcus'' are generally considered facultative anaerobes and oxygen usually does not negatively affect their growth, some strains may show less growth in the presence of oxygen and are considered anaerophilic, meaning that the presence of oxygen inhibits their growth (and therefore their acid production) but they can still grow in the presence of O<sub>2</sub>. The presence of CO<sub>2</sub> has a positive effect on acid production <ref name="NAKAGAWA"></ref><ref name="Oevelen_1979" />. Therefore, it is generally best practice to seal the starter with an airlock. | ||
+ | |||
+ | ====Starter Information==== | ||
+ | General starter information for commercial ''Pediococcus'' cultures is limited. In addition to the various [[Pediococcus#Manufacturer_Tips|starter suggestions by various labs]] regarding their individual cultures, Jeff Mello of Bootleg Biology reported good growth results when using 2 grams of calcium carbonate (chalk; CaCO3) per liter of wort for starters of Sour Weapon <ref name="mello_starter"></ref>. This same formula might benefit other ''Pediococcus'' starters as well. The CaCO3 serves as a buffering agent that keeps the starter pH from getting too low too fast, and is similar to the concept of buffered growth media for ''Lactobacillus'' (see [[Lactobacillus#Samuel_Aeschlimann.27s_Starter_Procedures|''Lactobacillus'' Starter]]). Starters of ''P. damnosus'' cultures could require around 84 hours for optimal growth, however, this time requirement hasn't been looked at in wort starters (only MRS media) <ref name="Nel"></ref>. | ||
+ | |||
+ | All species require nicotinic acid (niacin), pantothenic acid (vitamin B<sub>5</sub>), and biotin (vitamin B<sub>7</sub>) for growth, so these vitamins could be added to ''Pediococcus'' specific growth media. Other vitamins, such as riboflavin and pyridoxine, might stimulate growth for some strains of ''P. damnosus'' <ref name="Wade_2018" />. | ||
+ | |||
+ | * For information on mixed culture starters, see [[Mixed_Cultures#Starters_and_Other_Manufacturer_Tips|Mixed Culture Starters]]. | ||
+ | |||
+ | ====Sulfur Dioxide==== | ||
+ | Sulfur dioxide (SO<sub>2</sub>) in the form of potassium metabisulfite is often used in wine to limit microbiological growth of yeast and bacteria, and oxidation. SO<sub>2</sub> maintains an equilibrium between molecular SO<sub>2</sub>, bisulfite, and sulfite ions. The molecular SO<sub>2</sub> portion interacts with cell walls because it has no charge, while the rest of the SO<sub>2</sub> has less of an impact on yeast and bacteria cells (but can still have some inmpact on bacterial cells, depending on species). Below a pH of 3.5, molecular SO<sub>2</sub> is higher, but above a pH of 3.5 the SO<sub>2</sub> binds more into bisulfite and sulfite ions, rendering it less effective against microbes. SO<sub>2</sub> can also become bound to other compounds such as acetaldehyde, pyruvic acid, anthocyanins, glucose, and glacturonic acid, which also renders it less effective against microbes, although they can degrade and release molecular SO<sub>2</sub> as well <ref name="Wade_2018" />. | ||
+ | |||
+ | ''Pediococcus'' and other lactic acid bacteria have a wide range of resistance to SO<sub>2</sub> depending on species and strain. One study by Edwards and Jensen (1992) reported that ''P. parvulus'' was able to thrive in 20 mg/L of free SO<sub>2</sub> (0.39 mg/L molecular SO<sub>2</sub>), indicating that small amounts of SO<sub>2</sub> are not enough to inhibit ''Pediococcus''. Other studies have shown that different strains can be inhibited at concentrations between 100-256 mg/L total SO<sub>2</sub> <ref name="Wade_2018" />. | ||
+ | |||
+ | ====Hop Resistance==== | ||
+ | ''Pediococcus'' species and strains are generally resistant to hop compounds, and have been reported to grow in beer with at least 30 IBU <ref name="Geissler"></ref>, and together with ''Lactobacillus'' are reported to be responsible for ~70% of all beer spoilage incidents caused by microbes <ref name="Garcia-Garcia">[http://onlinelibrary.wiley.com/doi/10.1002/jib.397/abstract Pediococcus damnosus strains isolated from a brewery environment carry the horA gene. Jorge Hugo Garcia-Garcia, Luis Cástulo Damas-Buenrostro, Juan Carlos Cabada-Amaya, Myriam Elias-Santos, Benito Pereyra-Alférez. 2017. DOI: 10.1002/jib.397.]</ref>. It has been suggested by research that horizontal gene transfer (transfer of genetic material by means other than reproduction) allows ''Pediococcus'' species (and other LAB) to obtain the genes associated with resistance to hops (primarily multi-drug transporter "horA", along with "hitA", "horC", and "horB" <ref name="Garcia-Garcia" />). This has been thought to allow ''Pediococcus'' to adapt to living in beer <ref name="Garcia-Garcia" /><ref name="Snauwaert"></ref>. | ||
+ | |||
+ | While able to grow in the presence of hops, the presence of hops still inhibits ''P. damnosus''. For example, one study found that in the presence of 15 IBU, lactic acid production was reduced by ~82%. Exposure to 15 IBU also increased diacetyl production by 350%, while 2,3-pentanedione (buttery, nutty, toasted, caramellic, diacetyl and acetoin notes <ref>[http://www.thegoodscentscompany.com/data/rw1003991.html "acetyl propionyl ". The Good Scents Company. Retrieved 01/18/2017.]</ref>) was decreased by 25%. Interestingly, exposure to 3% ABV and no hops reduced the production of diacetyl and 2,3-pentanedione by about 20%. The addition of vitamins, specifically thiamine (vitamin B1) and riboflavin (vitamin B2), increased the production of lactic acid in the presence of 15 IBU or no hops by about 15-30%. However, thiamine also increased diacetyl production by 100-125% and 2,3-pentadione production by 20-30% without the presence of hops. In the presence of 15 IBU, thiamine and/or riboflavin increased diacetyl production by about 26-36% and 2,3-pentadione by about 40-114% <ref>[http://onlinelibrary.wiley.com/doi/10.1002/jib.385/full The influence of thiamine and riboflavin on various spoilage microorganisms commonly found in beer. Barry Hucker, Melinda Christophersen, Frank Vriesekoop. 2017.]</ref>. Strains that are grown exposed to small amounts of iso-alpha acids can be adapted to survive higher amounts up to ~150 μg mL<sup>1</sup>. The implications of this in brewing mean that the bacteria can enter any stage of the process and remain in undetectable amounts until it adapts to higher IBU conditions and then potentially spoils beer <ref name="Garcia-Garcia" />. | ||
+ | |||
+ | It was reported that ''Pedioccoccus pentosaceus'' can be inhibited by iso-alpha acids and beta acids (although beta acids are not soluble in beer or wort) and that the power of inhibition by these hop acids is greater in beer fermentation than it is on MRS media due to the lower pH of beer and the presence of ethanol. Hop acids, in general, lose their antimicrobial properties as the pH of their solution rises to around 5.5 <ref>[https://www.notulaebotanicae.ro/index.php/nbha/article/view/11687 Inhibitory Effects of Iso-α and β Hop Acids Against Pediococcus pentosaceus. Delia MICHIU, Frank DELVIGNE, Nicolas MABON, Mirela JIMBOREAN, Melinda FOGARASI, Mihaela MIHAI, Maria TOFANĂ, Philippe THONART. 2019.]</ref> | ||
+ | |||
+ | ====Mixed Culture Influence==== | ||
+ | |||
+ | See [[Lactic Acid]]. | ||
+ | |||
+ | ====VBNC==== | ||
+ | ''P. damnosus'' has been found to be able to enter a so-called "viable but nonculturable (VBNC) state" where cells are not culturable by routine means, but are still alive and recoverable when stress conditions are removed. This can lead to problems identifying these microorganisms in potentially spoiled products. See [https://www.sciencedirect.com/science/article/pii/S002364382101776X Zhenbo Xu et .al (2021)] for potential resuscitation strategies for ''P. damnosus''. | ||
+ | |||
+ | See also: | ||
+ | * [[Quality_Assurance#Viable_But_Nonculturable|Quality Assurance]] | ||
+ | |||
+ | ===Carbohydrate Metabolism=== | ||
+ | [[File:Pedio sugars.JPG|thumb|''Pediococcus'' fermentables based on species; table from [https://www.springer.com/us/book/9780387333410 "Wine Microbiology. Practical Applications and Procedures.", Kenneth C. Fugelsang, Charles G. Edwards, 2007.]]] | ||
+ | |||
+ | ''P. damnosus'' can ferment glucose, sucrose, and fructose. Some strains of ''P. damnosus'' can ferment maltose, sucrose, and galactose <ref name="ucdavis"></ref><ref name="Oevelen_1979" />. The disaccharide trehalose is the preferred carbon source for pediococci <ref name="Geissler"></ref>. While simple sugars are the primary food source for ''Pediococcus'', many strains of ''P. damnosus'' have been observed to produce varying degrees of both alpha and beta-glucosidase enzymes. Alpha-glucosidase enzymes have the ability to break down higher chain sugars, including dextrins, starches, and glucans (possibly even the glucans that are produced by ''P. damnosus'' that result in ropy beer). The types of beta-glucosidase enzymes produced by ''P. damnosus'' are thought to perhaps play a role in breaking down monoglycosidic bonds (see [[Glycosides]]), but cannot break down the more complex diglycosidic bonds which are needed to break down many glycosides that would release flavor and aroma compounds. Compared to the microbe ''Oenococcus oeni'' which is often used in wine and cider fermentation (malolactic fermentation) and has been shown to have more impactful beta-glucosidase activity, ''P. damnosus'' is thought to be less impactful on glycosides. Unlike ''O. oeni'' which decreases its enzymatic activity in low pH conditions, enzymatic activity of ''P. damnosus'' is very stable at a pH of 3-4. Very low concentrations of glucose or fructose (1 g/l) inhibit this enzymatic activity in ''P. damnosus''. The presence of alcohol inhibits the alpha-glucosidase activity in most strains, which might contribute to longer lasting ropiness in beer. The optimal temperature for enzymatic activity in ''P. damnosus'' is between 35-40°C (95-104°F) <ref>[http://onlinelibrary.wiley.com/doi/10.1111/j.1365-2672.2005.02707.x/full Screening of Lactobacillus spp. and Pediococcus spp. for glycosidase activities that are important in oenology. A. Grimaldi, E. Bartowsky, V. Jiranek. 2005. DOI: 10.1111/j.1365-2672.2005.02707.x.]</ref>. Some strains of ''P. damnosus'' found in lambic have been found to contain the genetic capability of breaking down ferulic acid into 4-vinylphenol, which can assist in ethyl phenol production later on by ''[[Brettanomyces]]'' <ref name="Roosa_2024">De Roos, J., Vermotea, L., Cnockaertb, M., Vandammeb, P., Weckxa, S., & De Vuysta, L. WOODEN BARRELS HELP TO STEER THE LAMBIC BEER FERMENTATION AND MATURATION PROCESS.</ref>. | ||
+ | |||
+ | ===Lactic Acid Production=== | ||
+ | [[File:Pedio EMP Pathway.jpg|thumb|200px|right|[https://onlinelibrary.wiley.com/doi/full/10.1111/ajgw.12366 Homofermentative Embden–Meyerhof–Parnas (EMP) pathway for the production of lactic acid, acetoin, diacetyl and 2,3‐butanediol and acetic acid production from citrate metabolism. Image by Wade et al (2018).]]] | ||
+ | |||
+ | About 90% of sugar metabolized by ''Pediococcus'' produces both L- and D-lactic acid <ref name="Wade_2018" />. It does so by homolactic fermentation producing primarily lactic acid (same EMP pathway as [[Lactobacillus#Types_of_Metabolism|''Lactobacillus'' homolactic fermentation]]), although some species/strains can convert glycerol to lactic acid, acetic acid, acetoin, and CO2 under aerobic conditions (''P. damnosus'' is not in this category) <ref>[https://books.google.com/books?id=1b1CAgAAQBAJ&pg=RA2-PA1&lpg=RA2-PA1&dq=pediococcus+damnosus+homolactic&source=bl&ots=myI2alVB78&sig=cG-yWB4GuABQFEtqD2CAyKmU0TE&hl=en&sa=X&ved=0CEAQ6AEwBGoVChMI66C5593-xgIVCVKICh3Pcg7c#v=onepage&q=pediococcus%20damnosus%20homolactic&f=false Encyclopedia of Food Microbiology. Pediococcus. Carl A. Batt. Academic Press, Sep 28, 1999 .]</ref>. Some strains of ''P. pentosaceus'' can ferment five-chain sugars such as xylose to produce acetic acid and lactic acid. Some strains of ''P. halophilus'' (now reclassified as ''Tetragenococcus halophilis'') can convert citric acid into acetic acid and oxaloacetate (oxaloacetate is then further reduced to acetic acid and diacetyl, and the diacetyl is further reduced to acetoin, and 2,3-butanediol) by producing citrate lyase enzyme. This generally occurs at a slower rate than malolactic fermentation and depends on pH and temperature. However, all species in the ''Pediococcus'' genus are considered obligatory homofermentative because of the pathways that they use <ref>[http://aem.asm.org/content/81/20/7233.full A Genomic View of Lactobacilli and pediococci Demonstrates that Phylogeny Matches Ecology and Physiology. Jinshui Zheng, Lifang Ruan, Ming Sun and Michael Gänzle. 2015.]</ref><ref name="Wade_2018" /><ref>[https://aem.asm.org/content/53/6/1257.short Citrate Metabolism by Pediococcus halophilus. Chiyuki Kanbe, Kinji Uchida. 1987.]</ref>. | ||
+ | |||
+ | ===Diacetyl and Acetoin=== | ||
+ | ''P. damnosus'' can produce high amounts of diacetyl and acetoin during lactic acid production <ref>[http://onlinelibrary.wiley.com/doi/10.1046/j.1365-2672.2000.00956.x/pdf Identification of pediococci by ribotyping. R. Satokari, T. Mattila-Sandholm and M.L. Suihko. Journal of Applied Microbiology 2000, 88, 260–265.]</ref><ref>[http://mmbr.asm.org/content/77/2/157.full The Microbiology of Malting and Brewing. Nicholas A. Bokulicha, and Charles W. Bamforth. June 2013.]</ref>. Diacetyl is the 'buttery' aroma and flavor found in beer (generally not favorable) and in wine (favorable in amounts between 1-4 mg/L). While other microbes found in beer and wine fermentation (namely ''Saccharomyces cerevisiae'') can also produce diacetyl, ''Pediococcus'' and other lactic acid bacteria are known to be able to produce much higher amounts <ref name="Wade_2018" />. | ||
+ | |||
+ | In lactic acid bacteria, diacetyl can be the byproduct of both homofermentative metabolism of sugars as well as the metabolism of citric acid, and it is a way for the cells to regenerate NADP<sup>+</sup>. In either of these two pathways, extra pyruvate is turned into alpha-acetolactate which then undergoes an oxidative decarboxylation reaction to produce diacetyl. Diacetyl is often reduced by yeast to acetoin and/or 2,3-butanediol, which have a higher threshold and less of an impact on the finished beer/wine <ref name="Wade_2018" />. In mixed fermentation sour beer, the breakdown of diacetyl into acetoin and 2,3-butanediol is often thought to be carried out by ''Brettanomyces'' in a similar way to ''Saccharomyces'' species, but this has not been investigated that we are aware of. It has been reported that diacetyl reduction is faster at a lower pH of around 3.5, which is a typical pH range for sour beer and might be one of the contributing factors to a lack of anecdotal reports of diacetyl in sour beer <ref>[https://onlinelibrary.wiley.com/doi/full/10.1002/jib.381 Michel, M., Meier‐Dörnberg, T., Jacob, F., Methner, F. ‐J., Wagner, R. S., and Hutzler, M. (2016) Review: Pure non‐Saccharomyces starter cultures for beer fermentation with a focus on secondary metabolites and practical applications. J. Inst. Brew., 122: 569– 587. doi: 10.1002/jib.381.]</ref><ref name="krogerus_2013">[https://www.researchgate.net/publication/259331290_125th_Anniversary_Review_Diacetyl_and_its_control_during_brewery_fermentation Krogerus, K. and Gibson, B.R. (2013), 125th Anniversary Review: Diacetyl and its control during brewery fermentation. J. Inst. Brew., 119: 86-97. https://doi.org/10.1002/jib.84.]</ref>. | ||
+ | |||
+ | Several variables the affect diacetyl and acetoin production have been identified. First of all, some strains of ''Pediococcus'' species produce diacetyl, and others do not. During malolactic fermentation (conversion of citric acid to lactic acid), the temperature at which MLF is conducted can influence whether or not diacetyl is produced. For example, two studies reported that more diacetyl and acetoin were produced during the MLF in wine at 18°C compared at 25°C. The effect of temperature is not well understood, but it has been hypothesized that it could be that at lower temperature yeast is less active and thus cannot break the diacetyl down to acetoin and 2,3-butanediol (extended time exposed to active yeast or on lees can reduce diacetyl in wine and probably also beer). If SO<sub>2</sub> is used, it can bind to diacetyl in a form that cannot be tasted, although the SO<sub>2</sub> can become unbound and release the diacetyl again. An increase in citric acid can also lead to more diacetyl under semi-aerobic conditions but not anaerobic conditions. The presence of glucose has also been associated with higher levels of diacetyl production in wine <ref name="Wade_2018" />. | ||
+ | |||
+ | ==="Ropy" or "Sick" Beer=== | ||
+ | [[File:Ropy example.jpg|thumb|300|Example of ropy beer brewed with The Yeast Bay Melange and dregs from Boon Mariage Parfait 2010. Photo is courtesy of Stuart Grant <ref>[https://www.facebook.com/groups/MilkTheFunk/permalink/1148859311808948/ Conversation with Stuart Grant on MTF. 09/16/2015.]</ref>]] | ||
+ | [[File:EPS.gif|thumb|300|Exopolysaccharide pathway <ref name="ESP">[http://www.sciencedirect.com/science/article/pii/S0740002004000668 Glucose fermentation kinetics and exopolysaccharide production by ropy Pediococcus damnosus IOEB8801. Emilie Walling, Marguerite Dols-Lafargue, Aline Lonvaud-Funel. Food Microbiology Volume 22, Issue 1, January 2005, Pages 71–78.]</ref>]] | ||
+ | |||
+ | Some strains of ''P. damnosus'' (and other bacteria) can cause a beer (or wine) to go "ropy", also known as "sick" by [[lambic]] brewers (or more specifically as "the fat sickness"; “la maladie de la graisse” in French <ref>[https://beerbybart.com/2011/04/03/true-lambic-jean-van-roy-cantillon-sick-beer/ True Lambic: Sick beers and the magic of Cantillon. Beer By Bart blog. Gail Ann Williams. 04/03/2011. Retrieved 04/23/2016.]</ref>). Reportedly, ropiness in beer that also has ''Brettanomyces'' (which is traditionally credited with breaking down the ropiness after a period of rest) usually lasts anywhere from 1 week to 3 months, although fewer reports claim that it has lasted as long as 7 months (see reference for different experiences of brewers) <ref name="ropy_time">[https://www.facebook.com/groups/MilkTheFunk/permalink/1132030550158491/ Poll on Milk The Funk regarding how long ropy beer has been observed. 08/20/2015.]</ref>. Some species of ''Pediococcus'' and other lactic acid bacteria have been reported to also be able to break down ropiness <ref>[https://www.facebook.com/groups/MilkTheFunk/permalink/1670311836330357/?comment_id=1670331339661740&comment_tracking=%7B%22tn%22%3A%22R0%22%7D Matt Humbard and Joe Idoni. Milk The Funk Facebook group. 04/29/2017.]</ref>. The viscosity of ropy beer has been reported to be higher at the bottom of wooden barrels than near the top, either due to sedimentation or perhaps more growth of ''Pediococcus'' near the bottom of wooden barrels due to yeast autolysis and/or less exposure to oxygen <ref name="Oevelen_1979" />. Despite the popularity of this talking point about ''Pediococcus'' in brewing, a lot of strains of ''Pediococcus'' used in brewing don't seem to produce ropiness, especially strains sourced from beer yeast labs. For example, Richard Preiss of [[Escarpment Laboratories]] reported only seeing ropiness from ''Pediococcus'' sourced from [[lambic]] <ref>[https://www.facebook.com/groups/MilkTheFunk/permalink/2859721020722760/?comment_id=2859868767374652&comment_tracking=%7B%22tn%22%3A%22R%22%7D Richard Preiss. Milk The Funk Facebook group thread on the frequency of ''Pediococcus'' caused ropiness. 08/20/2019.]</ref>. | ||
+ | |||
+ | [https://www.tandfonline.com/doi/abs/10.1094/ASBCJ-37-0034 Oevelen and Verachtert (1979)] demonstrated that ''Brettanommyces bruxllensis'' reduces the viscosity of ropiness produced by ''Pediococcus'', but that at least one strain of ''Saccharomyces cerevisiae'' does not <ref name="Oevelen_1979" />. The scientists tested two strains of ''B. bruxellensis'', and when either one of these strains were co-pitched with a strain of ''P. damnosus'' (formerly called ''P. cerevisiae''), at two weeks there was the same level of viscosity as when the ''P. damnosus'' strain was pitched by itself, but at 4 weeks the viscosity was greatly reduced (although the viscosity was still higher than when there was no ''Pediococcus'' present). When they co-pitched a strain of ''Saccharomyces cerevisiae'' with the ''Pediococcus'', the viscosity remained high at 4 weeks, demonstrating that this strain of ''S. cerervisiae'' was not able to reduce the viscosity of the ropiness (it is not known if this strain of ''S. cerevisiae'' was diastatic or not). The scientists also attempted to stagger the pitch of ''Brettanomyces'', pitching it two weeks after the ''Pediococcus''. This resulted in very low growth of the ''Brettanomyces'' and no reduction in viscosity due to that limited growth. The limited growth was attributed to the low pH that the ''Brettanomyces'' yeast was exposed to at inoculation time. The ''Brettanomyces'' was able to reduce the viscosity when the scientists add another 10 g/L of glucose, and buffered the pH to 4. The researchers also found that when ropy media was exposed to air, it immediately disappeared. However, exposing ropy beer to air is not an acceptable method for dealing with ropiness due to oxidation and acetic acid production in sour beer when it is exposed to oxygen <ref name="Oevelen_1979" />. The exact enzymatic activity for how ''Brettanomyces'' (or other microorganisms) break down EPS from ''Pediococcus'' is not well characterized, but it could be due to alpha-glucosidase activity in those yeasts (see [[Brettanomyces#Carbohydrate_Metabolism_and_Fermentation_Temperature|''Brettanomyces'' metabolism]] and [[Pediococcus#Carbohydrate_Metabolism|Carbohydrate Metabolism]] above). | ||
+ | |||
+ | This "ropiness" is caused by production of exopolysaccharides (EPS) in the form of β-glucans (beta glucans) by some strains ''Pediococcus'' and some other lactic acid bacteria species. Ropiness also contains 4-15% proteins and nucleic acid in the form of RNA <ref name="Oevelen_1979" />. The β-glucans are made up of beta 1, 3 linkages and beta 1, 2 branches composed of single units <ref name="Wade_2018" />. A small amount (20-30 mg/L <ref name="Wade_2018" />) of β-glucan is adequate enough to affect the visible viscosity of beer or wine. The gene known as "dps" has been identified with the production of β-glucan/EPS in ''P. damnosus'', and the gene "gtf" in ''P. claussenii'' <ref name="Snauwaert"></ref>. Not all strains of ''P. damnosus'' express the gene, and only ones that do will cause a beer to go ropy. Although it is not needed to survive in beer, EPS production is probably has importance in biofilm production <ref>[http://cat.inist.fr/?aModele=afficheN&cpsidt=23890699 Ethanol tolerance of lactic acid bacteria, including relevance of the exopolysaccharide gene gtf. Pittet V, Morrow K, Ziola B. 2011.]</ref>, and pediococci that are ropy have been found to be more acid, alcohol, and SO2 tolerant than other pediococci. The thickness of the ropiness is increased with the presence of malic acid <ref name="ESP"></ref>. While strains of ''P. damnosus'' and ''P. parvulus'' are the ''Pediococcus'' species most associated with ropiness, some strains of ''P. pentosaceus'' have also been found to produce EPS <ref name="Wade_2018" />. | ||
+ | |||
+ | One study showed that the production of β-glucan coincided with the end of the growth phase of ''Pediococcus''. While small amounts of β-glucan were produced during growth, after 2 days of growth, β-glucan production increased as growth slowed. β-glucan production stopped when growth stopped. This study showed that β-glucan production is linked to ''Pediococcous'' growth, producing more towards the end of growth. This study found that other variables were not factors in the production of β-glucan, such as differing levels of alcohol (although alcohol interacts with the β-glucan in a way that makes the viscosity seem thicker). The study also found that the lack of agitation increased the β-glucan production (wine makers will often agitate or aerate ropy wine to cure the wine from ropiness). A higher initial pH encourages higher growth (5.5+), which increases β-glucan production. A lower initial pH (3.5), decreases growth and β-glucan production. A higher concentration of glucose increased growth and β-glucan production. Glucose is needed for β-glucan production. While fructose alone is mostly insufficient to produce ropiness, a combination of glucose and fructose was slightly more efficient than glucose alone <ref name="ESP"></ref>. The introduction of malic acid, glucose, fructose, and/or nitrogen from things like fruit that is added to sour beer or even sugar added for natural carbonation can trigger ''Pediococcus'' growth and EPS production. | ||
+ | |||
+ | Temperature and nitrogen levels also affect how much EPS is produced. One study found that at 12°C both growth and EPS production was much slower than at 25°C. After 29 days in agar media, the EPS in the 12°C samples tended to reach or slightly exceed the levels in the 25°C samples, which developed equivilant levels of EPS (or slightly less) within 7-13 days. Nitrogen levels also play a significant role, according to this study, particularly at lower fermentation temperatures. At 12°C, nitrogen was more important for the formation of EPS than glucose (although glucose was found to be the most important factor in EPS development overall, which is in agreement with the previously cited study). At 25°C nitrogen levels played a significant role in producing EPS, however less so than glucose levels. In general though, higher availability of nitrogen complimented higher levels of glucose to produce more EPS (and faster/higher cell growth) <ref>[https://www.sciencedirect.com/science/article/pii/S0168160503000606 Exopolysaccharide production by Pediococcus damnosus 2.6 in a semidefined medium under different growth conditions. Maite Dueñas, Arantza Munduate, Aidé Perea, Ana Irastorza. 2003.]</ref>. Stress in the environment such as ethanol and SO<sub>2</sub> has also been shown to induce EPS production, and a lack of available glucose has been associated with eliminating the production of EPS (e.g. malolactic fermentation by ''Pediococcus'' in wine tends not to produce EPS, perhaps due to the lack of glucose in the environment) <ref name="Wade_2018" />. | ||
+ | |||
+ | The presence of beta-glucans from barley have been observed to extend both the growth and the viability of ''Lactobacillus'' species in probiotics, indicating that ropiness might be a stress response <ref>[http://www.mdpi.com/1422-0067/15/2/3025/htm Barley β-Glucans-Containing Food Enhances Probiotic Performances of Beneficial Bacteria. Mattia P. Arena, Graziano Caggianiello, Daniela Fiocco, Pasquale Russo, Michele Torelli, Giuseppe Spano, and Vittorio Capozzi. 2014.]</ref><ref>[http://www.mdpi.com/1422-0067/13/5/6026/htm Beta-Glucans Improve Growth, Viability and Colonization of Probiotic Microorganisms. Pasquale Russo, Paloma López, Vittorio Capozzi, Pilar Fernández de Palencia, María Teresa Dueñas, Giuseppe Spano, and Daniela Fiocco. 2012.]</ref>. One study looked at this effect in beta-glucans produced by ''Pediococcus parvulus'' and found that ''L. plantarum'' had a longer viability in a fermented medium with no additional food source when that medium was first fermented with ''P. parvulus'' and EPS was produced. The ''L. plantarum'' strain that was tested did not ferment the beta-glucans. This suggests that there is an interspecies simbiotic relationship between lactic acid bacteria that produce EPS and those that don't, and when EPS is produced (beta-glucans are present) the bacteria survive longer. The study also observed that more EPS was produced in an oat based wort and a rice based wort, while no EPS was produced in a barley based wort, suggesting that different food sources influence whether or not EPS is produced <ref>[http://www.mdpi.com/1422-0067/18/7/1588/htm In Situ β-Glucan Fortification of Cereal-Based Matrices by Pediococcus parvulus. Adrián Pérez-Ramos, María Luz Mohedano, Paloma López, Giuseppe Spano, Daniela Fiocco, Pasquale Russo, and Vittorio Capozzi. 2017.]</ref>. Pittet et al. (2011) found that the presence of the EPS genes in lactic acid bacteria did not correspond with the ability to survive beer-level ethanol levels or higher, which led to the hypothesis that perhaps EPS provides LAB a way to assist in the formation of [[Quality_Assurance#Biofilms|biofilm]], although this has yet to be demonstrated scientifically <ref>[https://www.tandfonline.com/doi/abs/10.1094/ASBCJ-2011-0124-01 Ethanol Tolerance of Lactic Acid Bacteria, Including Relevance of the Exopolysaccharide Gene Gtf. Vanessa Pittet and Kendra Morrow and Barry Ziola. 2011. DOI: https://doi.org/10.1094/ASBCJ-2011-0124-01.]</ref>. Along these same lines, [https://www.academia.edu/27927065/Lysozyme_resistance_of_the_ropy_strain_Pediococcus_parvulus_IOEB_8801_is_correlated_with_beta_glucan_accumulation_around_the_cell Coulon et al. (2012)] reported that an EPS producing strain of ''Pediococcus parvulus'' was more resistant to the antimicrobial enzyme [https://en.wikipedia.org/wiki/Lysozyme lysozyme], which is often added to wine to kill lactic acid bacteria. The beta-glucan EPS formed a "coat" around the cells of the bacteria, thus protecting it from the lysozyme enzyme. When a beta-glucanase enzyme was added to break down the EPS produced by the bacteria, the beta-glucan "coat" disappeared from the cell walls and the lysozyme was once again effective at killing this strain of ''Pediococcus'' <ref>[https://www.academia.edu/27927065/Lysozyme_resistance_of_the_ropy_strain_Pediococcus_parvulus_IOEB_8801_is_correlated_with_beta_glucan_accumulation_around_the_cell Coulon, Joana et al. “Lysozyme Resistance of the Ropy Strain Pediococcus Parvulus IOEB 8801 Is Correlated with Beta-Glucan Accumulation around the Cell.” International Journal of Food Microbiology 159.1 (2012): 25–29. Web.]</ref>. | ||
+ | |||
+ | It has been observed that ''Lactobacillus'' species can produce EPS (''Lactococcus lactis'', ''Lactobacillus delbrueckii'', ''Lactobacillus casei'', and ''Lactobacillus helveticus'') <ref name="ESP"></ref>. Some ''Oenococcus oeni'' strains can also produce EPS <ref>[https://pubmed.ncbi.nlm.nih.gov/19659698/ Ciezack G, Hazo L, Chambat G, Heyraud A, Lonvaud-Funel A, Dols-Lafargue M. Evidence for exopolysaccharide production by Oenococcus oeni strains isolated from non-ropy wines. J Appl Microbiol. 2010 Feb;108(2):499-509. doi: 10.1111/j.1365-2672.2009.04449.x. Epub 2009 Jun 30. PMID: 19659698.</ref>. Some species of yeast can also produce EPS, including ''Candida'', ''Cryptococcus'', ''Debaryomyces'', ''Lipomyces'', ''Pichia'' <ref>[https://www.facebook.com/groups/MilkTheFunk/permalink/2465980936763439/ Zach Taggart. Milk the Funk Facebook group post on EPS from yeast. 01/16/2019.]</ref>, ''Pseudozyma'', ''Rhodotorula'' and ''Sporobolomyces'' <ref name="Gientka_2015">[https://www.researchgate.net/publication/283498621_Exopolysaccharides_from_yeast_insight_into_optimal_conditions_for_biosynthesis_chemical_composition_and_functional_properties_-_review?fbclid=IwAR1X6Y0rnquoF6SD-eH9m6EWpLIefgZJFUJK51NJYBooJWngxEVS2aR3PKE Exopolysaccharides from yeast: insight into optimal conditions for biosynthesis, chemical composition and functional properties - review. Iwona Gientka, Stanisław Błażejak, Stanisław Błażejak, Lidia Stasiak, Lidia Stasiak, Anna Chlebowska-Śmigiel, Anna Chlebowska-Śmigiel. 2015.]</ref>. | ||
+ | |||
+ | ====Videos==== | ||
+ | * [https://www.facebook.com/groups/MilkTheFunk/permalink/4547361381958707/ Highly viscous ropy beer video posted in MTF by Roi Funk Krispen.] | ||
+ | * [https://www.facebook.com/groups/MilkTheFunk/permalink/3478055812222608/ Post on MTF by Brandon Jones showing initial ropiness of a beer, and then after aging the ropiness out for 8 weeks.] | ||
+ | * [https://www.facebook.com/groups/MilkTheFunk/permalink/1813022388725967/ Other videos on MTF of ropy beer.] | ||
+ | |||
+ | ===Bacteriocins=== | ||
+ | Some strains of ''Pediococcus pentocaseus'' have been found to produce bacteriocins, which are toxins that are produced by the cells that inhibit the growth of other types of bacteria. For example, Bootleg Biology's "Sour Weapon" culture produces antimicrobials called bacteriocins or pediocins (see Bootleg Biology's [https://www.facebook.com/BootlegBiology/photos/a.148869931970401.1073741829.124634287727299/465185997005458/?type=1&theater Facebook post] regarding bacteriocins for more info). These can inhibit and kill similar species of bacteria like ''Lactobacillus'' and other ''Pediococcus'' species in mixed-culture fermentations. Bacteriocin production can be higher when ''Pediococcus pentocaseus'' is co-fermented with other bacteria than when it is fermented on its own <ref>[https://www.frontiersin.org/articles/10.3389/fmicb.2018.02952/abstract Enhanced Bacteriocin Production by Pediococcus pentosaceus 147 in Co-Culture with Lactobacillus plantarum LE27 on Cheese Whey Broth. Carolina Gutiérrez-Cortés, Héctor Suarez, Gustavo Buitrago, Luis A. Nero and Svetoslav D. Todorov. 2018. DOI: 10.3389/fmicb.2018.02952.]</ref>. A strain of ''Pediococcus pentocaseus'' was found to produce a "class II" enteriocin that inhibits the growth of ''E. coli'' and ''Staphylococcus aureus'' <ref>[https://www.sciencedirect.com/science/article/pii/S0882401017314559 Antibacterial activity of Lactobacillus plantarum isolated from Tibetan yaks. Lei Wang, Hui Zhang, Mujeeb Ur Rehman, Khalid Mehmood, Xiong Jiang, Mujahid Iqbal, Xiaole Tong, Xing Gao, Jiakui Li. 2017.]</ref>. ''P. damnosus'' also produces an antimicrobial compound called pediocin PD-1, which can inhibit several bacteria spp including ''O. oeni'' <ref>[http://onlinelibrary.wiley.com/doi/10.1046/j.1365-2672.2001.01486.x/full Growth optimization of Pediococcus damnosus NCFB 1832 and the influence of pH and nutrients on the production of pediocin PD-1. H.A. Nel1, R. Bauer, E.J. Vandamme and L.M.T. Dicks. Jan 2002.]</ref><ref>[http://www.ncbi.nlm.nih.gov/pubmed/15878403 Purification, partial amino acid sequence and mode of action of pediocin PD-1, a bacteriocin produced by Pediococcus damnosus NCFB 1832. Bauer R, Chikindas ML, Dicks LM. May 2005.]</ref>. | ||
+ | |||
+ | See also: | ||
+ | * [[Lactobacillus#Bacteriocins|''Lactobacillus'' bacteriocins]]. | ||
+ | |||
+ | ===Malolactic Fermentation=== | ||
+ | See the [[Cider#Microbes|Cider]] page. | ||
+ | |||
+ | ===Biogenic Amines=== | ||
+ | Biogenic amines are produced by all living things and are present in many fermented beverages. High dosages can lead to health issues such as vomiting, headache, asthma, hypotension, and cardiac palpitation. Thus, biogenic amines have been studied intensely <ref name="Wade_2018" />. For more information on biogenic amines in beer in general, see [http://suigenerisbrewing.com/index.php/2019/01/22/biogenic-amines/ "Fact or Fiction – Biogenic Amines in Beer" by Dr. Bryan Heit]. | ||
+ | |||
+ | Some strains of lactic acid bacteria, including ''Pediococcus'', can metabolize amino acids into biogenic amines, and potentially also degrade them <ref>[https://watermark.silverchair.com/0362-028x-60_7_831.pdf Tyramine Formation by Pediococcus spp. during Beer Fermentation. MARIA IZQUlERDO-PULIDO, JOSEP-MIQUEL CARCELLER-ROSA, ABEL MARINE-FONT, and M. CARMEN VIDAL-CAROU. 1996.]</ref><ref>[https://www.researchgate.net/publication/240429641_Effect_of_tyrosin_on_tyramin_formation_during_beer_fermentation Effect of tyrosin on tyramin formation during beer fermentation. Maria Izquierdo-Pulido, M. Carmen Vidal-Carou. 2000.]</ref>. The number of strains capable of producing biogenic amines appears to be very low. [https://link.springer.com/pdf%2F10.1007%2FBF01105812 Weiller and Radler (1976)] found that only one out 28 strains of ''P. cerevisiae'' (later reclassified to ''P. damnosus'' and ''P. pentosaceus'') produced biogenic amines. [https://www.ncbi.nlm.nih.gov/pubmed/973463 Strickland et al. (2016)] found that out of multiple species of ''Pediococcus'', only one strain of ''P. inopinatus'' produced biogenic amines, and it only produced 3.3 mg/L of histamine. [https://www.sciencedirect.com/science/article/pii/S0168160511002893 García‐Ruiz et al. (2011)] reported that 9 out of 85 strains of ''P. parvulus'' and ''P. pentosaceus'' were able to degrade some biogenic amines (histamine, tyrosine, and putrescine) in culture media, but were unable to do so in wine, indicating that any degradation of biogenic amines in wine that might occur is not likely due to lactic acid bacteria, but due to some other cause <ref name="Wade_2018" />. | ||
+ | |||
+ | Izquierdo-Pulido et al. (1995) found that out of 35 samples of Spanish lagers contaminated with ''Pediococcus'', 21 of them had final tyramine levels between 5-10 mg/l, 6 of them had no detected tyramine, and 8 of them had high levels around 25 mg/l, with higher levels being correlated to higher cell counts of ''Pediococcus''. higher levels of tyramine were associated with higher cell counts of the tyramine-producing strains during smaller bench test fermentations as well. There was no correlation between the presence of wild yeast and tyramine production. Filtration and pasteurization after fermentation had no effect on the levels of tyramine in the final beers <ref>[https://watermark.silverchair.com/0362-028x-59_2_175.pdf Biogenic Amine Changes Related to Lactic Acid Bacteria During Brewing. MARIAI ZQUIERDO-PULIDO, JUDIT FONT-FABREGAS, JOSEP-MIQUEL CARCELLER-ROSA, ABEL MARINE-FONT, and CARMENVIDAL-CAROU. 1995.]</ref>. | ||
+ | |||
+ | De Roos et al (2024) found the ''P. damnosus'' in two samples of lambic had the genetic capability to produce histamine. The total biogenic amine content of the lambic was at levels below that which has been deemed safe by European regulatory bodies <ref name="Roosa_2024"/>. | ||
+ | |||
+ | See also: | ||
+ | * [[Spontaneous_Fermentation#Biogenic_Amines|Biogenic amines in spontaneously fermented beer.]] | ||
+ | * [[Brettanomyces#Biogenic_Amines|Biogenic amine production by ''Brettanomyces''.]] | ||
+ | * [[Wine#Biogenic_Amines|Biogenic amines in wine.]] | ||
+ | * [http://www.horscategoriebrewing.com/2019/01/spontaneous-fermentation-and-biogenic.html "Spontaneous fermentation and biogenic amines" by Dr. Dave Janssen; review of several studies that looked at the levels of biogenic amines in different beers and their production, and their potential flavor contribution.] | ||
+ | * [http://suigenerisbrewing.com/index.php/2019/01/22/biogenic-amines/ "Fact or Fiction – Biogenic Amines in Beer" by Dr. Bryan Heit; an analysis of biogenic amines in spontaneously fermented beer and associated health concerns.] | ||
+ | ** [https://www.facebook.com/groups/MilkTheFunk/permalink/2570617069633158/?comment_id=2570641549630710&reply_comment_id=2570664762961722&comment_tracking=%7B%22tn%22%3A%22R8%22%7D Follow up from Dr. Heit on MTF on "allergic-like" reactions to biogenic amines.] | ||
+ | |||
+ | ===Other Metabolites=== | ||
+ | ''P. claussenii'' tends to produce a smaller amount of acetic acid than lactic acid in about a 1:3 ratio. ''P. damnosus'' tends to produce only lactic acid and no acetic acid <ref name="Geissler">[http://www.sciencedirect.com/science/article/pii/S0168160515301033 Metabolic strategies of beer spoilage lactic acid bacteria in beer. Andreas J. Geissler, Jürgen Behr, Kristina von Kamp, Rudi F. Vogel. 2015.]</ref>, although some strains have been found to produce small amounts of acetic acid of around 100-300 ppm. This level is slightly below and above flavor thresholds in lager beer (but could be additive with other organisms) <ref>[http://onlinelibrary.wiley.com/doi/10.1002/j.2050-0416.2010.tb00393.x/abstract Isolation, Identification, and Characterisation of Beer-Spoilage Lactic Acid Bacteria from Microbrewed Beer from Victoria, Australia. Garry Menz, Christian Andrighetto, Angiolella Lombardi, Viviana Corich, Peter Aldred, Frank Vriesekoop. 2010.]</ref>, but significantly less than the total acetic acid often found in gueuze (around 700-2200 ppm <ref>[http://www.horscategoriebrewing.com/2016/07/duivelsbier-of-halle.html Jansen, Dave. Hors Category Blog. "Duivelsbier of Halle". 07/30/2016. Retrieved 01/31/2018.]</ref>) and Flanders reds (300-2300 ppm <ref>[http://www.sciencedirect.com/science/article/pii/S0168160515301896 Microbial diversity and metabolite composition of Belgian red-brown acidic ales. Isabel Snauwaert, Sanne P. Roels, Filip Van Nieuwerburg, Anita Van Landschoot, Luc De Vuyst, Peter Vandamme. 2015.]</ref>). ''Pediococcus'' also carries the decarboxylase enzyme (PAD) which converts hydroxycinnamic acid (ferulic acid) into phenols (4-vinyl guaiacol) <ref name="lentz_2018">[http://www.mdpi.com/2311-5637/4/1/20/html#B13-fermentation-04-00020 The Impact of Simple Phenolic Compounds on Beer Aroma and Flavor. Michael Lentz. 2018. doi: 10.3390/fermentation4010020.]</ref>. | ||
+ | |||
+ | While ''Pediococcus'' has been linked to the creation of acrolein via the metabolism of glycerol, evidence supports that this is very rare in ''Pediococcus'' species. In wine, acrolein reacts with anthocyanins and other phenols to produce an intense bitterness. Acrolein production in wine has been linked to some strains of ''Lactobacillus''. Some strains of ''Pediococcus'' have been found to metabolize gylcerol in beer and wine under aerobic and semi-aerobic environments, but the metabolism of glycerol in the presence of oxygen results in the production of lactic acid, acetic acid, diacetyl, and 2,3-butanediol, and not acrolein <ref name="Wade_2018" />. | ||
+ | |||
+ | ==Storage== | ||
+ | For instructions on how to make slants at home capable of storing any microbe for potentially 2+ years, [http://suigenerisbrewing.blogspot.com/2015/11/easy-home-yeast-banking-and-video.html see Bryan's video on Sui Generis Brewing (requires a pressure cooker)]. | ||
+ | |||
+ | Justin Amaral recommends using isotonic sodium chloride and freezing media that is meant for 32°F freezers (lasts around 2 years) <ref>[https://www.facebook.com/groups/MilkTheFunk/permalink/1859788084049397/?comment_id=1859790364049169&comment_tracking=%7B%22tn%22%3A%22R0%22%7D Justin Amaral. Milk The Funk Facebook comment on storing ''Pediococcus''. 10/23/2017.]</ref>. | ||
+ | |||
+ | ==See Also== | ||
+ | ===Additional Articles on MTF Wiki=== | ||
+ | * [[Alternative Bacteria Sources]] | ||
+ | * [[Spontaneous Fermentation]] | ||
+ | * [[Mixed Fermentation]] | ||
+ | * [[Mixed Cultures]] | ||
+ | * [[Scientific Publications]] | ||
+ | * [[Lactobacillus]] | ||
+ | * [[Glycosides]] | ||
− | + | ===External Resources=== | |
+ | * [http://wineserver.ucdavis.edu/industry/enology/winemicro/winebacteria/pediococcus_damnosus.html UC Davis article stub on Pediococcus damnosus.] | ||
+ | * [https://books.google.com/books?id=hPjpBwAAQBAJ&printsec=frontcover#v=onepage&q&f=false The Genera of Lactic Acid Bacteria, by W.H.N Holzapfel, Brian J.B. Wood. Partially free.] | ||
+ | * [https://www.facebook.com/groups/MilkTheFunk/permalink/1247801211914757/?comment_id=1247830631911815&comment_tracking=%7B%22tn%22%3A%22R0%22%7D MTF Thread with Bryan from Sui Generis on suggestions/theories on selecting for ''Pediococcus'' on plates.] | ||
+ | * [https://www.youtube.com/watch?v=a--DVtJtU4A Dan Pixley's comparison between White Labs, Wyeast, and Inland Island ''Pediococcus'' cultures.] | ||
==References== | ==References== |
Latest revision as of 16:16, 6 April 2024
Pediococcus (often referred to by brewers as "Pedio") are Gram-positive lactic acid bacteria (LAB) used in the production of Belgian style beers where additional acidity is desirable. They are native to plant material and fruits [1], and often found in spontaneously fermented beer as the primary source of lactic acid production (with P. damnosus being the only species identified in Lambic) [2][3]. It is also seen as a major source of beer contamination in commercial breweries due to its ability to adapt to and survive in beer. The ability to grow in beer is strain dependent rather than species dependent, however, genetic differences indicate that P. damnosus and P. claussenii are better adapted to surviving in beer than P. pentosaceus [4]. Like many bacteria, pediococci have the ability to transfer genes horizontally without reproduction [4]. They are generally considered to be facultative anaerobes, which means they grow anaerobically but can also grow in the presence of oxygen [5]. Some species/strains (including individual strains of P. damnosus) can have their growth and acid production inhibited by oxygen [6], while some will have better growth and produce more acid in the presence of oxygen (microaerophilic) [7][8]. Strains found in beer are hop tolerant [9]. Due to their continued metabolism of longer chain polysaccharides, acid production will increase with storage time. Pediococcus can form a pellicle.
Pediococcus may also cause “ropiness” (also called a "sick beer") due to the production of exopolysaccharides when exposed to a fresh sugar source. "Ropy" or "sick" beer is more viscous and, in extreme circumstances, can form strands. Sickness effects mostly the mouthfeel and appearance of the beer, and may have no influence on the flavor. It is considered a temporary flaw in sour beer. Some brewers, including Vinnie Cilurzo from Russian River Brewing and some Belgian lambic producers, claim that after the ropiness goes away (generally in 3-6 months [10]) it produces a deeper acidity and mouthfeel, and is viewed as a positive process in the production of sour beer [11]. For other brewers, ropy beer is seen as a nuisance due to the beer needing to be aged for a longer period of time, especially when it occurs shortly after bottling. Pediococcus species can also produce diacetyl with extended storage time [12]. Brettanomyces can break down exopolysaccharides and diacetyl produced by Pediococcus and the two are often used together.
See Lactobacillus, Brettanomyces, Saccharomyces, Mixed Cultures, Kveik, and Nonconventional Yeasts and Bacteria charts for other commercially available cultures.
Contents
- 1 Introduction of Characteristics and Taxonomy
- 2 Commercial Pediococcus Cultures
- 3 Metabolism
- 4 Storage
- 5 See Also
- 6 References
Introduction of Characteristics and Taxonomy
Pediococcus can either improve or detract from fermented food products, including beer, wine, and cider. They play an important role in the fermentation of some meats and cheeses. They tend to be the primary souring microorganism in the production of Belgian lambic. Some species have also been isolated from plants, fruit, and fermenting plant material. In wine, P. parvulus is the most common species found, and in beer P. damnosus is the most common. P. pentosaceus tends to be more hop sensitive than P. damnosus [13].
Currently, there are 11 recognized species of Pediococcus. They are P. acidilactici, P. argentinicus, P. cellicola, P. claussenii, P. damnosus, P. ethanolidurans, P. inopinatus, P. parvulus, P. pentosaceus (subspecies pentosaceus and intermedius), P. siamensis, and P. stilesii. P. cerevisiae was reclassified into either P. damnosus or P. pentosaceus. Other species of Pediococcus have also been reclassified to other genera in the last couple of decades. P. dextrinicus is now classified as Lactobacillus dextrinicus, P. urinae-equi is now classified as Aerococcus urinae‐equi, and P. halophilis is now classified as Tetragenococcus halophilis [13].
Pediococci are described as coccoidal (spherical) or ovoid (egg-shaped) in shape. They are Gram-positive, non-motile (not capable of moving on their own), and non-spore forming. They are obligate homofermentive and typically do not produce CO2, ethanol, or acetic acid, although there are a few exceptions to this in the literature. They do not produce catalase (except for some P. pentocaseus strains which were reported to have pseudo-catalase activity by Simpson and Taguchi 1995) or oxidase enzymes. Because of the way that Pedioccous cells divide, they often appear stuck together in pairs or clumps. They are the only lactic acid bacteria found in wine and beer to do this, so they are easily identifiable at the genus level under a microscope based on their tendency to clump together. When grown on agar that is supplemented with 100 mg/L of pimaricin, the colonies are white-grey with a diameter of about 1 mm. Ropy strains have a high elasticity, and when touched with a needle, long threads can be drawn and sometimes the colonies completely stick to the needle. See table 2 from Wade et al. (2018) for more species identification indicators and what carbohydrates different species can ferment [13][14].
See also:
Commercial Pediococcus Cultures
Name | Mfg# | Taxonomy | Note |
---|---|---|---|
Bootleg Biology/Spot Yeast | Sour Weapon P (Pediococcus pentosaceus Blend) | P. pentosaceus blend | Perfect for acidifying unhopped wort quickly for kettle or “quick” sours. At 98F, it’s capable of achieving a pH of 3.3 within 18 hours. At 84F, it can reach a pH of 3.5 within 24 hours. With more time, a terminal pH of 3.1 may be reached. P. pentosaceus can also be used for long-term sours. It is capable of growing and producing lactic acid in worts with IBUs as high as 30, though it is recommended for unhopped worts as IBUs over 10 may prevent significant quick souring. At ~30 IBU, souring occurs in 2-3 months [15]. This culture may produce antimicrobials called bacteriocins or pediocins. These can inhibit and kill similar species of bacteria like Lactobacillus and other Pediococcus species in mixed-culture fermentations. Read Bootleg Biology's Facebook post regarding bacteriocins for more info. No signs of ropiness (exopolysaccharides) have occurred in testing [16]. It is still unknown how hops will affect souring in a long term scenario. Bootleg Biology is still researching long term effects and awaiting peoples feedback as of 5/23/2016. |
East Coast Yeast | ECY33 | P. parvulus | Isolated from lambic which was refermented with grapes, this strain of Pediococcus produces lactic acid, diacetyl, and may cause ropiness in beer. Always add Brettanomyces where Pediococcus is used [17]. |
Escarpment Laboratories | Pediococcus Blend | Two Pediococcus strains (P. damnosus and P. pentosaceus) | This blend of 2 hop-resistant Pediococcus strains (P. damnosus and P. pentosaceus) is intended for use in long-term souring. Neither strain has been observed to produce exopolysaccharides (EPS). We recommend 15 IBU or less in the first generation. |
Inland Island Yeast Laboratories | INISBC-998 | P. damnosus | Gram positive cocci that produces lactic acid. Also produces diacetyl and several proteins that may cause a "rope" to form in the beer. Rope will disappear with time. Oxygen and hop sensitive. 75-90°F Temperature Range. No longer available. |
Mainiacal Yeast (CLOSED) | MYPP1 | P. pentocaceus | Isolated from a growing marijuana plant. No production of diacetyl or EPS, with a clean acidity. Ferment at 70-100°F [18]. Commercial pitches only. |
Mainiacal Yeast (CLOSED) | MYPD1 | P. damnosus | Isolated from a bottle of belgian lambic. Produces diacetyl and EPS, so use in conjunction with Brettanomyces. Ferment at 70-90°F [18]. Commercial pitches only. |
Omega Yeast Labs | OYL-606 | P. damnosus | This modestly hop tolerant Pedio strain produces a clean lactic tang over time. The strain can produce diacetyl so it is often paired with one or more Brett strains (to consume the diacetyl). While more hop tolerant than the Lacto Blend (OYL-605), IBUs over 5-10 IBU may inhibit souring. Souring time can vary depending on IBU level [19]. EPS production is unknown and has not been observed or reported [20]. Commercial pitches only. |
Propagate Lab | MIP-920 | P. damnosus | Will sour a finished beer over time to a pH of 3.5 - 3.1. It works well at room temperature and is hop tolerant. It may produce ropiness [21]. |
RVA Yeast Labs | RVA 601 | P. damnosus | Lactic acid bacteria used in souring Belgian-style beers such as gueze and Lambic. Acid production increases with storage. Temperature range is 60-95°F. |
White Labs | WLP661 | P. damnosus | High diacetyl (a.k.a. butter) producer and slow growing. Fermentation temp: 70-85°F (21-29.4° C). Attenuation: 65%. Cell count: 50-80 million cells/mL [22]. Homebrew vials contain 35 mL of slurry, and ~1.75-2.8 billion cells per vial [23]. |
Wyeast | 5733 | P. damnosus (previously listed as P. cerevisiae) | May cause “ropiness” and produce low levels of diacetyl with extended storage time. Temp range: 60-95° F (15-35° C). Cell count: 1.0 x 108 (100 million) cells/mL [24]. Homebrew packs contain 100-125 mL of slurry, and ~10-12.5 billion cells [23]. |
Manufacturer Tips
Bootleg Biology on Sour Weapon P
This product, which is a blend of Pediococcus pentosaceus, can be used for a kettle souring process or a mixed fermentation process. Jeff reported good growth results using 2 grams of calcium carbonate (chalk; CaCO3) per liter of wort for starters of Sour Weapon [25]. This same formula might benefit other Pediococcus starters as well. The CaCO3 serves as a buffering agent that keeps the starter pH from getting too low too fast, and is similar to the concept of buffered growth media for Lactobacillus (see Lactobacillus Starter).
Regarding exposure to oxygen in general:
"We don't propagate Sour Weapon on plates or at the liquid stage anaerobically, and definitely don't have problems with growth or souring. We have not tested pitching the culture in aerated wort, but there's certainly the chance that you could be increasing the likelihood of off flavors. Sour Weapon does still work very well when co-pitched with yeast in wort that has been aerated though. Hope that helps." - Jeff Mello of Bootleg Biology [26].
Wyeast on 5733
"If using 1 pack of 5733 per 5 gallon batch; and either adding to secondary after alcoholic fermentation is complete, or co-inoculating with a Saccharomyces' strain, then a starter would not be necessary. If you did want or need to propagate, I'd recommend 2 liters of 1.030-1.040 wort per pack, incubated at 80-90*F, without agitation." - Michael Dawson, Wyeast.
"For propagation, we recommend using 1.040 OG wort and incubating at 30-35*C without aeration for 48-96 hours; pH drop will indicate when it's ready to pitch. For co-inoculation or post-primary addition, we recommend 0.5 million cells per mL, which is the equivalent of 1 pack in 5 gallons/20 liters. For larger volumes, you can propagate and inoculate with the starter culture at a rate 2.5-5% of the total volume of the main batch." - Michael Dawson, Wyeast.
White Labs on WLP661
"That one does well in 70-85 deg F. You can do a starter, but you shouldn't have to if you are doing a 5 gallon batch. It does take a while to sour, so just be patient and let it do it's thing." Sarah Neel, Sales and Customer Service, White Labs
Tips From MTF
The Rare Barrel
"We just performed an interesting experiment at The Rare Barrel with pedio. Jay, our head brewer and blender, wanted a more acidic beer that we could use as a blending component while also growing up our diminishing pedio culture. So we racked 2 oak barrels of gold fermented with Brettanomyces claussenii and White Labs Pediococcus damnosus (WLP661) into one of our 30 bbl batches of 12*p gold wort that had been acidified to about 4.5 pH in the kettle using lactic acid (our first hot side experiment!). No oxygen, really wanted to encourage the bacteria. Within 10 days the pH was 3.6 and the gravity 10.7. We were all surprised how quickly the pedio was working. We eventually racked a "splash" of fermenting gold with BSI Brett D and BSI Lacto D to drop the gravity. The beer had a bright acidity quickly and I was surprised at how well rounded the flavors were when we racked into barrels after a month in the fermenting vessel.
This might be a little harder to do at home, but I think there's potential for interesting results. Pediococcus shines long term traditionally so I agree with the posts above, if you're going for quick acidity I'd go lacto, but I plan on playing around with early Pediococcus fermentations at home." - Mike Makris from The Rare Barrel [27].
Pediococcus has a reputation for being a "slow worker", meaning that it produces acidity overtime. Staff at The Rare Barrel noticed that pitching on second generations of White Labs WLP661 Pediococcus might help the Pediococcus produce acidity very quickly [28] (~19 minutes in). They speculate that oxygen exposure might inhibit acid production with the White Labs WLP661 P. damnosus strain, however this is purely speculative and anecdotal [28] (~32 minutes in).
Metabolism
Growth and Environment
Pediococcus generally has a high tolerance to ethanol compared to other bacteria, and can range from 10-25% ABV, depending on the species and strain. P. damnosus is sensitive to warm temperatures. It is unable to grow at 35°C or higher. The optimal growth occurs at 22°C. Other species can be more tolerant of higher temperatures, for example, P. parvulus and P. inopinatus have been shown to grow between 12 to 40°C, with rapid death occuring at 45°C [13]. P. damnosus is sensitive to environments that contain NaCl, and will not grow with concentrations of 4% NaCl [1]. Most strains of P. claussenii can grow in beer. About half of the strains tested of P. damnosus can grow in beer, and none to very few strains of P. acidilactici, P. parvulus, and P. pentosaceus have been found to grow in finished beer.
Another environmental factor that can affect the growth and survival of Pediococcus is pH. P. damnosus is unable to grow at a pH of 8 or higher. One study showed that optimal growth for P. damnosus was observed in MRS media after ~84 hours with an initial pH of 6.7, and a final pH of 4.14, which occurred naturally from fermentation. The addition of bacteriological peptone, MnSO4, and Tween 80 also increased activity. Maximum cell densities of P. damnosus are around 4.3 billion cells/mL in MRS media starting at a pH of 5.5 [29][30], but this is only in optimal conditions. Maximum cell density varies based on the conditions of the propagation with pH and nutrient demands being two of the main limiting factors [31].
Although more experiments are probably needed, agitation is believed to be an important factor for any species of microbe (yeast and bacteria). Gentle stirring on a stir plate or orbital shaker, or frequent gentle manual agitation leads to faster growth and a higher number of organisms. Agitation keeps the microbes in solution. It also maximizes the microbes' access to nutrients and disperses waste evenly. In a non-agitated starter, the microbes are limited to the diffusion rate of nutrients, leading to a slower and more stressful growth [32]. Although Pediococcus are generally considered facultative anaerobes and oxygen usually does not negatively affect their growth, some strains may show less growth in the presence of oxygen and are considered anaerophilic, meaning that the presence of oxygen inhibits their growth (and therefore their acid production) but they can still grow in the presence of O2. The presence of CO2 has a positive effect on acid production [6][14]. Therefore, it is generally best practice to seal the starter with an airlock.
Starter Information
General starter information for commercial Pediococcus cultures is limited. In addition to the various starter suggestions by various labs regarding their individual cultures, Jeff Mello of Bootleg Biology reported good growth results when using 2 grams of calcium carbonate (chalk; CaCO3) per liter of wort for starters of Sour Weapon [25]. This same formula might benefit other Pediococcus starters as well. The CaCO3 serves as a buffering agent that keeps the starter pH from getting too low too fast, and is similar to the concept of buffered growth media for Lactobacillus (see Lactobacillus Starter). Starters of P. damnosus cultures could require around 84 hours for optimal growth, however, this time requirement hasn't been looked at in wort starters (only MRS media) [30].
All species require nicotinic acid (niacin), pantothenic acid (vitamin B5), and biotin (vitamin B7) for growth, so these vitamins could be added to Pediococcus specific growth media. Other vitamins, such as riboflavin and pyridoxine, might stimulate growth for some strains of P. damnosus [13].
- For information on mixed culture starters, see Mixed Culture Starters.
Sulfur Dioxide
Sulfur dioxide (SO2) in the form of potassium metabisulfite is often used in wine to limit microbiological growth of yeast and bacteria, and oxidation. SO2 maintains an equilibrium between molecular SO2, bisulfite, and sulfite ions. The molecular SO2 portion interacts with cell walls because it has no charge, while the rest of the SO2 has less of an impact on yeast and bacteria cells (but can still have some inmpact on bacterial cells, depending on species). Below a pH of 3.5, molecular SO2 is higher, but above a pH of 3.5 the SO2 binds more into bisulfite and sulfite ions, rendering it less effective against microbes. SO2 can also become bound to other compounds such as acetaldehyde, pyruvic acid, anthocyanins, glucose, and glacturonic acid, which also renders it less effective against microbes, although they can degrade and release molecular SO2 as well [13].
Pediococcus and other lactic acid bacteria have a wide range of resistance to SO2 depending on species and strain. One study by Edwards and Jensen (1992) reported that P. parvulus was able to thrive in 20 mg/L of free SO2 (0.39 mg/L molecular SO2), indicating that small amounts of SO2 are not enough to inhibit Pediococcus. Other studies have shown that different strains can be inhibited at concentrations between 100-256 mg/L total SO2 [13].
Hop Resistance
Pediococcus species and strains are generally resistant to hop compounds, and have been reported to grow in beer with at least 30 IBU [33], and together with Lactobacillus are reported to be responsible for ~70% of all beer spoilage incidents caused by microbes [12]. It has been suggested by research that horizontal gene transfer (transfer of genetic material by means other than reproduction) allows Pediococcus species (and other LAB) to obtain the genes associated with resistance to hops (primarily multi-drug transporter "horA", along with "hitA", "horC", and "horB" [12]). This has been thought to allow Pediococcus to adapt to living in beer [12][4].
While able to grow in the presence of hops, the presence of hops still inhibits P. damnosus. For example, one study found that in the presence of 15 IBU, lactic acid production was reduced by ~82%. Exposure to 15 IBU also increased diacetyl production by 350%, while 2,3-pentanedione (buttery, nutty, toasted, caramellic, diacetyl and acetoin notes [34]) was decreased by 25%. Interestingly, exposure to 3% ABV and no hops reduced the production of diacetyl and 2,3-pentanedione by about 20%. The addition of vitamins, specifically thiamine (vitamin B1) and riboflavin (vitamin B2), increased the production of lactic acid in the presence of 15 IBU or no hops by about 15-30%. However, thiamine also increased diacetyl production by 100-125% and 2,3-pentadione production by 20-30% without the presence of hops. In the presence of 15 IBU, thiamine and/or riboflavin increased diacetyl production by about 26-36% and 2,3-pentadione by about 40-114% [35]. Strains that are grown exposed to small amounts of iso-alpha acids can be adapted to survive higher amounts up to ~150 μg mL1. The implications of this in brewing mean that the bacteria can enter any stage of the process and remain in undetectable amounts until it adapts to higher IBU conditions and then potentially spoils beer [12].
It was reported that Pedioccoccus pentosaceus can be inhibited by iso-alpha acids and beta acids (although beta acids are not soluble in beer or wort) and that the power of inhibition by these hop acids is greater in beer fermentation than it is on MRS media due to the lower pH of beer and the presence of ethanol. Hop acids, in general, lose their antimicrobial properties as the pH of their solution rises to around 5.5 [36]
Mixed Culture Influence
See Lactic Acid.
VBNC
P. damnosus has been found to be able to enter a so-called "viable but nonculturable (VBNC) state" where cells are not culturable by routine means, but are still alive and recoverable when stress conditions are removed. This can lead to problems identifying these microorganisms in potentially spoiled products. See Zhenbo Xu et .al (2021) for potential resuscitation strategies for P. damnosus.
See also:
Carbohydrate Metabolism
P. damnosus can ferment glucose, sucrose, and fructose. Some strains of P. damnosus can ferment maltose, sucrose, and galactose [1][14]. The disaccharide trehalose is the preferred carbon source for pediococci [33]. While simple sugars are the primary food source for Pediococcus, many strains of P. damnosus have been observed to produce varying degrees of both alpha and beta-glucosidase enzymes. Alpha-glucosidase enzymes have the ability to break down higher chain sugars, including dextrins, starches, and glucans (possibly even the glucans that are produced by P. damnosus that result in ropy beer). The types of beta-glucosidase enzymes produced by P. damnosus are thought to perhaps play a role in breaking down monoglycosidic bonds (see Glycosides), but cannot break down the more complex diglycosidic bonds which are needed to break down many glycosides that would release flavor and aroma compounds. Compared to the microbe Oenococcus oeni which is often used in wine and cider fermentation (malolactic fermentation) and has been shown to have more impactful beta-glucosidase activity, P. damnosus is thought to be less impactful on glycosides. Unlike O. oeni which decreases its enzymatic activity in low pH conditions, enzymatic activity of P. damnosus is very stable at a pH of 3-4. Very low concentrations of glucose or fructose (1 g/l) inhibit this enzymatic activity in P. damnosus. The presence of alcohol inhibits the alpha-glucosidase activity in most strains, which might contribute to longer lasting ropiness in beer. The optimal temperature for enzymatic activity in P. damnosus is between 35-40°C (95-104°F) [37]. Some strains of P. damnosus found in lambic have been found to contain the genetic capability of breaking down ferulic acid into 4-vinylphenol, which can assist in ethyl phenol production later on by Brettanomyces [38].
Lactic Acid Production
About 90% of sugar metabolized by Pediococcus produces both L- and D-lactic acid [13]. It does so by homolactic fermentation producing primarily lactic acid (same EMP pathway as Lactobacillus homolactic fermentation), although some species/strains can convert glycerol to lactic acid, acetic acid, acetoin, and CO2 under aerobic conditions (P. damnosus is not in this category) [39]. Some strains of P. pentosaceus can ferment five-chain sugars such as xylose to produce acetic acid and lactic acid. Some strains of P. halophilus (now reclassified as Tetragenococcus halophilis) can convert citric acid into acetic acid and oxaloacetate (oxaloacetate is then further reduced to acetic acid and diacetyl, and the diacetyl is further reduced to acetoin, and 2,3-butanediol) by producing citrate lyase enzyme. This generally occurs at a slower rate than malolactic fermentation and depends on pH and temperature. However, all species in the Pediococcus genus are considered obligatory homofermentative because of the pathways that they use [40][13][41].
Diacetyl and Acetoin
P. damnosus can produce high amounts of diacetyl and acetoin during lactic acid production [42][43]. Diacetyl is the 'buttery' aroma and flavor found in beer (generally not favorable) and in wine (favorable in amounts between 1-4 mg/L). While other microbes found in beer and wine fermentation (namely Saccharomyces cerevisiae) can also produce diacetyl, Pediococcus and other lactic acid bacteria are known to be able to produce much higher amounts [13].
In lactic acid bacteria, diacetyl can be the byproduct of both homofermentative metabolism of sugars as well as the metabolism of citric acid, and it is a way for the cells to regenerate NADP+. In either of these two pathways, extra pyruvate is turned into alpha-acetolactate which then undergoes an oxidative decarboxylation reaction to produce diacetyl. Diacetyl is often reduced by yeast to acetoin and/or 2,3-butanediol, which have a higher threshold and less of an impact on the finished beer/wine [13]. In mixed fermentation sour beer, the breakdown of diacetyl into acetoin and 2,3-butanediol is often thought to be carried out by Brettanomyces in a similar way to Saccharomyces species, but this has not been investigated that we are aware of. It has been reported that diacetyl reduction is faster at a lower pH of around 3.5, which is a typical pH range for sour beer and might be one of the contributing factors to a lack of anecdotal reports of diacetyl in sour beer [44][45].
Several variables the affect diacetyl and acetoin production have been identified. First of all, some strains of Pediococcus species produce diacetyl, and others do not. During malolactic fermentation (conversion of citric acid to lactic acid), the temperature at which MLF is conducted can influence whether or not diacetyl is produced. For example, two studies reported that more diacetyl and acetoin were produced during the MLF in wine at 18°C compared at 25°C. The effect of temperature is not well understood, but it has been hypothesized that it could be that at lower temperature yeast is less active and thus cannot break the diacetyl down to acetoin and 2,3-butanediol (extended time exposed to active yeast or on lees can reduce diacetyl in wine and probably also beer). If SO2 is used, it can bind to diacetyl in a form that cannot be tasted, although the SO2 can become unbound and release the diacetyl again. An increase in citric acid can also lead to more diacetyl under semi-aerobic conditions but not anaerobic conditions. The presence of glucose has also been associated with higher levels of diacetyl production in wine [13].
"Ropy" or "Sick" Beer
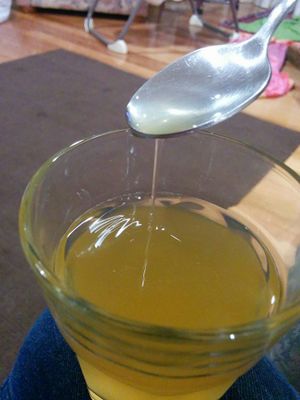
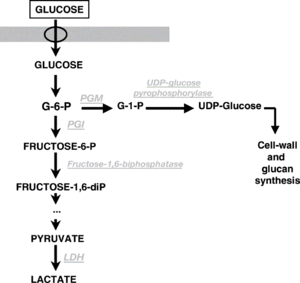
Some strains of P. damnosus (and other bacteria) can cause a beer (or wine) to go "ropy", also known as "sick" by lambic brewers (or more specifically as "the fat sickness"; “la maladie de la graisse” in French [48]). Reportedly, ropiness in beer that also has Brettanomyces (which is traditionally credited with breaking down the ropiness after a period of rest) usually lasts anywhere from 1 week to 3 months, although fewer reports claim that it has lasted as long as 7 months (see reference for different experiences of brewers) [10]. Some species of Pediococcus and other lactic acid bacteria have been reported to also be able to break down ropiness [49]. The viscosity of ropy beer has been reported to be higher at the bottom of wooden barrels than near the top, either due to sedimentation or perhaps more growth of Pediococcus near the bottom of wooden barrels due to yeast autolysis and/or less exposure to oxygen [14]. Despite the popularity of this talking point about Pediococcus in brewing, a lot of strains of Pediococcus used in brewing don't seem to produce ropiness, especially strains sourced from beer yeast labs. For example, Richard Preiss of Escarpment Laboratories reported only seeing ropiness from Pediococcus sourced from lambic [50].
Oevelen and Verachtert (1979) demonstrated that Brettanommyces bruxllensis reduces the viscosity of ropiness produced by Pediococcus, but that at least one strain of Saccharomyces cerevisiae does not [14]. The scientists tested two strains of B. bruxellensis, and when either one of these strains were co-pitched with a strain of P. damnosus (formerly called P. cerevisiae), at two weeks there was the same level of viscosity as when the P. damnosus strain was pitched by itself, but at 4 weeks the viscosity was greatly reduced (although the viscosity was still higher than when there was no Pediococcus present). When they co-pitched a strain of Saccharomyces cerevisiae with the Pediococcus, the viscosity remained high at 4 weeks, demonstrating that this strain of S. cerervisiae was not able to reduce the viscosity of the ropiness (it is not known if this strain of S. cerevisiae was diastatic or not). The scientists also attempted to stagger the pitch of Brettanomyces, pitching it two weeks after the Pediococcus. This resulted in very low growth of the Brettanomyces and no reduction in viscosity due to that limited growth. The limited growth was attributed to the low pH that the Brettanomyces yeast was exposed to at inoculation time. The Brettanomyces was able to reduce the viscosity when the scientists add another 10 g/L of glucose, and buffered the pH to 4. The researchers also found that when ropy media was exposed to air, it immediately disappeared. However, exposing ropy beer to air is not an acceptable method for dealing with ropiness due to oxidation and acetic acid production in sour beer when it is exposed to oxygen [14]. The exact enzymatic activity for how Brettanomyces (or other microorganisms) break down EPS from Pediococcus is not well characterized, but it could be due to alpha-glucosidase activity in those yeasts (see Brettanomyces metabolism and Carbohydrate Metabolism above).
This "ropiness" is caused by production of exopolysaccharides (EPS) in the form of β-glucans (beta glucans) by some strains Pediococcus and some other lactic acid bacteria species. Ropiness also contains 4-15% proteins and nucleic acid in the form of RNA [14]. The β-glucans are made up of beta 1, 3 linkages and beta 1, 2 branches composed of single units [13]. A small amount (20-30 mg/L [13]) of β-glucan is adequate enough to affect the visible viscosity of beer or wine. The gene known as "dps" has been identified with the production of β-glucan/EPS in P. damnosus, and the gene "gtf" in P. claussenii [4]. Not all strains of P. damnosus express the gene, and only ones that do will cause a beer to go ropy. Although it is not needed to survive in beer, EPS production is probably has importance in biofilm production [51], and pediococci that are ropy have been found to be more acid, alcohol, and SO2 tolerant than other pediococci. The thickness of the ropiness is increased with the presence of malic acid [47]. While strains of P. damnosus and P. parvulus are the Pediococcus species most associated with ropiness, some strains of P. pentosaceus have also been found to produce EPS [13].
One study showed that the production of β-glucan coincided with the end of the growth phase of Pediococcus. While small amounts of β-glucan were produced during growth, after 2 days of growth, β-glucan production increased as growth slowed. β-glucan production stopped when growth stopped. This study showed that β-glucan production is linked to Pediococcous growth, producing more towards the end of growth. This study found that other variables were not factors in the production of β-glucan, such as differing levels of alcohol (although alcohol interacts with the β-glucan in a way that makes the viscosity seem thicker). The study also found that the lack of agitation increased the β-glucan production (wine makers will often agitate or aerate ropy wine to cure the wine from ropiness). A higher initial pH encourages higher growth (5.5+), which increases β-glucan production. A lower initial pH (3.5), decreases growth and β-glucan production. A higher concentration of glucose increased growth and β-glucan production. Glucose is needed for β-glucan production. While fructose alone is mostly insufficient to produce ropiness, a combination of glucose and fructose was slightly more efficient than glucose alone [47]. The introduction of malic acid, glucose, fructose, and/or nitrogen from things like fruit that is added to sour beer or even sugar added for natural carbonation can trigger Pediococcus growth and EPS production.
Temperature and nitrogen levels also affect how much EPS is produced. One study found that at 12°C both growth and EPS production was much slower than at 25°C. After 29 days in agar media, the EPS in the 12°C samples tended to reach or slightly exceed the levels in the 25°C samples, which developed equivilant levels of EPS (or slightly less) within 7-13 days. Nitrogen levels also play a significant role, according to this study, particularly at lower fermentation temperatures. At 12°C, nitrogen was more important for the formation of EPS than glucose (although glucose was found to be the most important factor in EPS development overall, which is in agreement with the previously cited study). At 25°C nitrogen levels played a significant role in producing EPS, however less so than glucose levels. In general though, higher availability of nitrogen complimented higher levels of glucose to produce more EPS (and faster/higher cell growth) [52]. Stress in the environment such as ethanol and SO2 has also been shown to induce EPS production, and a lack of available glucose has been associated with eliminating the production of EPS (e.g. malolactic fermentation by Pediococcus in wine tends not to produce EPS, perhaps due to the lack of glucose in the environment) [13].
The presence of beta-glucans from barley have been observed to extend both the growth and the viability of Lactobacillus species in probiotics, indicating that ropiness might be a stress response [53][54]. One study looked at this effect in beta-glucans produced by Pediococcus parvulus and found that L. plantarum had a longer viability in a fermented medium with no additional food source when that medium was first fermented with P. parvulus and EPS was produced. The L. plantarum strain that was tested did not ferment the beta-glucans. This suggests that there is an interspecies simbiotic relationship between lactic acid bacteria that produce EPS and those that don't, and when EPS is produced (beta-glucans are present) the bacteria survive longer. The study also observed that more EPS was produced in an oat based wort and a rice based wort, while no EPS was produced in a barley based wort, suggesting that different food sources influence whether or not EPS is produced [55]. Pittet et al. (2011) found that the presence of the EPS genes in lactic acid bacteria did not correspond with the ability to survive beer-level ethanol levels or higher, which led to the hypothesis that perhaps EPS provides LAB a way to assist in the formation of biofilm, although this has yet to be demonstrated scientifically [56]. Along these same lines, Coulon et al. (2012) reported that an EPS producing strain of Pediococcus parvulus was more resistant to the antimicrobial enzyme lysozyme, which is often added to wine to kill lactic acid bacteria. The beta-glucan EPS formed a "coat" around the cells of the bacteria, thus protecting it from the lysozyme enzyme. When a beta-glucanase enzyme was added to break down the EPS produced by the bacteria, the beta-glucan "coat" disappeared from the cell walls and the lysozyme was once again effective at killing this strain of Pediococcus [57].
It has been observed that Lactobacillus species can produce EPS (Lactococcus lactis, Lactobacillus delbrueckii, Lactobacillus casei, and Lactobacillus helveticus) [47]. Some Oenococcus oeni strains can also produce EPS [58]. Some species of yeast can also produce EPS, including Candida, Cryptococcus, Debaryomyces, Lipomyces, Pichia [59], Pseudozyma, Rhodotorula and Sporobolomyces [60].
Videos
- Highly viscous ropy beer video posted in MTF by Roi Funk Krispen.
- Post on MTF by Brandon Jones showing initial ropiness of a beer, and then after aging the ropiness out for 8 weeks.
- Other videos on MTF of ropy beer.
Bacteriocins
Some strains of Pediococcus pentocaseus have been found to produce bacteriocins, which are toxins that are produced by the cells that inhibit the growth of other types of bacteria. For example, Bootleg Biology's "Sour Weapon" culture produces antimicrobials called bacteriocins or pediocins (see Bootleg Biology's Facebook post regarding bacteriocins for more info). These can inhibit and kill similar species of bacteria like Lactobacillus and other Pediococcus species in mixed-culture fermentations. Bacteriocin production can be higher when Pediococcus pentocaseus is co-fermented with other bacteria than when it is fermented on its own [61]. A strain of Pediococcus pentocaseus was found to produce a "class II" enteriocin that inhibits the growth of E. coli and Staphylococcus aureus [62]. P. damnosus also produces an antimicrobial compound called pediocin PD-1, which can inhibit several bacteria spp including O. oeni [63][64].
See also:
Malolactic Fermentation
See the Cider page.
Biogenic Amines
Biogenic amines are produced by all living things and are present in many fermented beverages. High dosages can lead to health issues such as vomiting, headache, asthma, hypotension, and cardiac palpitation. Thus, biogenic amines have been studied intensely [13]. For more information on biogenic amines in beer in general, see "Fact or Fiction – Biogenic Amines in Beer" by Dr. Bryan Heit.
Some strains of lactic acid bacteria, including Pediococcus, can metabolize amino acids into biogenic amines, and potentially also degrade them [65][66]. The number of strains capable of producing biogenic amines appears to be very low. Weiller and Radler (1976) found that only one out 28 strains of P. cerevisiae (later reclassified to P. damnosus and P. pentosaceus) produced biogenic amines. Strickland et al. (2016) found that out of multiple species of Pediococcus, only one strain of P. inopinatus produced biogenic amines, and it only produced 3.3 mg/L of histamine. García‐Ruiz et al. (2011) reported that 9 out of 85 strains of P. parvulus and P. pentosaceus were able to degrade some biogenic amines (histamine, tyrosine, and putrescine) in culture media, but were unable to do so in wine, indicating that any degradation of biogenic amines in wine that might occur is not likely due to lactic acid bacteria, but due to some other cause [13].
Izquierdo-Pulido et al. (1995) found that out of 35 samples of Spanish lagers contaminated with Pediococcus, 21 of them had final tyramine levels between 5-10 mg/l, 6 of them had no detected tyramine, and 8 of them had high levels around 25 mg/l, with higher levels being correlated to higher cell counts of Pediococcus. higher levels of tyramine were associated with higher cell counts of the tyramine-producing strains during smaller bench test fermentations as well. There was no correlation between the presence of wild yeast and tyramine production. Filtration and pasteurization after fermentation had no effect on the levels of tyramine in the final beers [67].
De Roos et al (2024) found the P. damnosus in two samples of lambic had the genetic capability to produce histamine. The total biogenic amine content of the lambic was at levels below that which has been deemed safe by European regulatory bodies [38].
See also:
- Biogenic amines in spontaneously fermented beer.
- Biogenic amine production by Brettanomyces.
- Biogenic amines in wine.
- "Spontaneous fermentation and biogenic amines" by Dr. Dave Janssen; review of several studies that looked at the levels of biogenic amines in different beers and their production, and their potential flavor contribution.
- "Fact or Fiction – Biogenic Amines in Beer" by Dr. Bryan Heit; an analysis of biogenic amines in spontaneously fermented beer and associated health concerns.
Other Metabolites
P. claussenii tends to produce a smaller amount of acetic acid than lactic acid in about a 1:3 ratio. P. damnosus tends to produce only lactic acid and no acetic acid [33], although some strains have been found to produce small amounts of acetic acid of around 100-300 ppm. This level is slightly below and above flavor thresholds in lager beer (but could be additive with other organisms) [68], but significantly less than the total acetic acid often found in gueuze (around 700-2200 ppm [69]) and Flanders reds (300-2300 ppm [70]). Pediococcus also carries the decarboxylase enzyme (PAD) which converts hydroxycinnamic acid (ferulic acid) into phenols (4-vinyl guaiacol) [71].
While Pediococcus has been linked to the creation of acrolein via the metabolism of glycerol, evidence supports that this is very rare in Pediococcus species. In wine, acrolein reacts with anthocyanins and other phenols to produce an intense bitterness. Acrolein production in wine has been linked to some strains of Lactobacillus. Some strains of Pediococcus have been found to metabolize gylcerol in beer and wine under aerobic and semi-aerobic environments, but the metabolism of glycerol in the presence of oxygen results in the production of lactic acid, acetic acid, diacetyl, and 2,3-butanediol, and not acrolein [13].
Storage
For instructions on how to make slants at home capable of storing any microbe for potentially 2+ years, see Bryan's video on Sui Generis Brewing (requires a pressure cooker).
Justin Amaral recommends using isotonic sodium chloride and freezing media that is meant for 32°F freezers (lasts around 2 years) [72].
See Also
Additional Articles on MTF Wiki
- Alternative Bacteria Sources
- Spontaneous Fermentation
- Mixed Fermentation
- Mixed Cultures
- Scientific Publications
- Lactobacillus
- Glycosides
External Resources
- UC Davis article stub on Pediococcus damnosus.
- The Genera of Lactic Acid Bacteria, by W.H.N Holzapfel, Brian J.B. Wood. Partially free.
- MTF Thread with Bryan from Sui Generis on suggestions/theories on selecting for Pediococcus on plates.
- Dan Pixley's comparison between White Labs, Wyeast, and Inland Island Pediococcus cultures.
References
- ↑ 1.0 1.1 1.2 Viticulture & Enology. UC Davis website. Pedioccous damnosus. Retrieved 07/28/2015.
- ↑ The Microbial Diversity of Traditional Spontaneously Fermented Lambic Beer. Freek Spitaels, Anneleen D. Wieme, Maarten Janssens, Maarten Aerts, Heide-Marie Daniel, Anita Van Landschoot, Luc De Vuyst, Peter Vandamme. April 18, 2014.
- ↑ Multiple Scientific publications linked on MTF.
- ↑ 4.0 4.1 4.2 4.3 Comparative genome analysis of Pediococcus damnosus LMG 28219, a strain well-adapted to the beer environment. Isabel Snauwaert, Pieter Stragier, Luc De Vuyst and Peter Vandamme. 2015.
- ↑ Lactic Acid Bacteria. Todar's Online Texbook of Bacteriology. Kenneth Todar, PhD. Pg 1. Retrieved 08/09/2015.
- ↑ 6.0 6.1 TAXONOMIC STUDIES ON THE GENUS PEDIOCOCCUS. ATSUSHI NAKAGAWA, KAKUO KITAHARA. 1959.
- ↑ THE NUTRITION AND PHYSIOLOGY OF THE GENUS PEDIOCOCCUS. Erling M. Jensen and Harry W. Seeley. 1954.
- ↑ Pediocins: The bacteriocins of pediococci. Sources, production, properties and applications. Maria Papagianni and Sofia Anastasiadou. 2009.
- ↑ Comparative genome analysis of Pediococcus damnosus LMG 28219, a strain well-adapted to the beer environment. Isabel Snauwaert, Pieter Stragier, Luc De Vuyst and Peter Vandamme. April 2015.
- ↑ 10.0 10.1 Poll on Milk The Funk regarding how long ropy beer has been observed. 08/20/2015.
- ↑ Cilurzo, Vinnie. AHA Sour Beer presentation. 2007.
- ↑ 12.0 12.1 12.2 12.3 12.4 Pediococcus damnosus strains isolated from a brewery environment carry the horA gene. Jorge Hugo Garcia-Garcia, Luis Cástulo Damas-Buenrostro, Juan Carlos Cabada-Amaya, Myriam Elias-Santos, Benito Pereyra-Alférez. 2017. DOI: 10.1002/jib.397.
- ↑ 13.00 13.01 13.02 13.03 13.04 13.05 13.06 13.07 13.08 13.09 13.10 13.11 13.12 13.13 13.14 13.15 13.16 13.17 13.18 Role of Pediococcus in winemaking. M.E. Wade, M.T. Strickland, J.P. Osborn, C.G. Edwards. 2018. DOI: https://doi.org/10.1111/ajgw.12366.
- ↑ 14.0 14.1 14.2 14.3 14.4 14.5 14.6 D. Van Oevelen & H. Verachtert (1979) Slime Production by Brewery Strains of Pediococcus Cerevisiae, Journal of the American Society of Brewing Chemists, 37:1, 34-37, DOI: 10.1094/ASBCJ-37-0034.
- ↑ Justin Amaral and Per Karlsson on Bootleg biology Sour Weapon hop tolerance. milk The Funk Facebook group. 11/2/2017.
- ↑ Bootleg Biology website. Retrieved 05/06/2016.
- ↑ "Wild Yeast / Brettanomyces / Lactic Bacteria". East Coast Yeast website. Retrieved 04/27/2018.
- ↑ 18.0 18.1 Private correspondence with Justin Amaral by Dan Pixley. 01/24/2018.
- ↑ Pediococcus OYL-606. Omega Yeast Labs website. Retrieved 06/11/2019.
- ↑ Adi Hastings. Milk The Funk Facebook group on OYL Pediococcus EPS production. 06/11/2019.
- ↑ Propagate Labs website. MIP-920. Retrieved 06/20/2020.
- ↑ Private correspondence with White Labs Customer Service and Dan Pixley. 10/29/2015.
- ↑ 23.0 23.1 Conversation with Richard Priess regarding WL and Wyeast homebrew pitches. 10/30/2015.
- ↑ Wyeast Specifications 2015 Retail Products. 2015.
- ↑ 25.0 25.1 Conversation on MTF with Jeff Mello regarding starters for Pediococcus. 08/08/2016.
- ↑ Discussion with Jeff Mello on MTF regarding Sour Weapon and oxygen. 09/27/2016.
- ↑ Conversation with Mike Makris on Milk The Funk.
- ↑ 28.0 28.1 The Sour Hour Episode 29 on The Brewing Network, interview with The Rare Barrel staff. 02/19/2016.
- ↑ Conversation with Richard Preiss on MTF regarding Pediococcus cell density. 07/19/2016.
- ↑ 30.0 30.1 Growth optimization of Pediococcus damnosus NCFB 1832 and the influence of pH and nutrients on the production of pediocin PD-1. Nel HA, Bauer R, Vandamme EJ, Dicks LM. 2001.
- ↑ Neva Parker, Reddit thread. 10/29/2015.
- ↑ Conversation with Bryan of Sui Generis Blog about starters and agitation. 11/09/2015.
- ↑ 33.0 33.1 33.2 Metabolic strategies of beer spoilage lactic acid bacteria in beer. Andreas J. Geissler, Jürgen Behr, Kristina von Kamp, Rudi F. Vogel. 2015.
- ↑ "acetyl propionyl ". The Good Scents Company. Retrieved 01/18/2017.
- ↑ The influence of thiamine and riboflavin on various spoilage microorganisms commonly found in beer. Barry Hucker, Melinda Christophersen, Frank Vriesekoop. 2017.
- ↑ Inhibitory Effects of Iso-α and β Hop Acids Against Pediococcus pentosaceus. Delia MICHIU, Frank DELVIGNE, Nicolas MABON, Mirela JIMBOREAN, Melinda FOGARASI, Mihaela MIHAI, Maria TOFANĂ, Philippe THONART. 2019.
- ↑ Screening of Lactobacillus spp. and Pediococcus spp. for glycosidase activities that are important in oenology. A. Grimaldi, E. Bartowsky, V. Jiranek. 2005. DOI: 10.1111/j.1365-2672.2005.02707.x.
- ↑ 38.0 38.1 De Roos, J., Vermotea, L., Cnockaertb, M., Vandammeb, P., Weckxa, S., & De Vuysta, L. WOODEN BARRELS HELP TO STEER THE LAMBIC BEER FERMENTATION AND MATURATION PROCESS.
- ↑ Encyclopedia of Food Microbiology. Pediococcus. Carl A. Batt. Academic Press, Sep 28, 1999 .
- ↑ A Genomic View of Lactobacilli and pediococci Demonstrates that Phylogeny Matches Ecology and Physiology. Jinshui Zheng, Lifang Ruan, Ming Sun and Michael Gänzle. 2015.
- ↑ Citrate Metabolism by Pediococcus halophilus. Chiyuki Kanbe, Kinji Uchida. 1987.
- ↑ Identification of pediococci by ribotyping. R. Satokari, T. Mattila-Sandholm and M.L. Suihko. Journal of Applied Microbiology 2000, 88, 260–265.
- ↑ The Microbiology of Malting and Brewing. Nicholas A. Bokulicha, and Charles W. Bamforth. June 2013.
- ↑ Michel, M., Meier‐Dörnberg, T., Jacob, F., Methner, F. ‐J., Wagner, R. S., and Hutzler, M. (2016) Review: Pure non‐Saccharomyces starter cultures for beer fermentation with a focus on secondary metabolites and practical applications. J. Inst. Brew., 122: 569– 587. doi: 10.1002/jib.381.
- ↑ Krogerus, K. and Gibson, B.R. (2013), 125th Anniversary Review: Diacetyl and its control during brewery fermentation. J. Inst. Brew., 119: 86-97. https://doi.org/10.1002/jib.84.
- ↑ Conversation with Stuart Grant on MTF. 09/16/2015.
- ↑ 47.0 47.1 47.2 47.3 Glucose fermentation kinetics and exopolysaccharide production by ropy Pediococcus damnosus IOEB8801. Emilie Walling, Marguerite Dols-Lafargue, Aline Lonvaud-Funel. Food Microbiology Volume 22, Issue 1, January 2005, Pages 71–78.
- ↑ True Lambic: Sick beers and the magic of Cantillon. Beer By Bart blog. Gail Ann Williams. 04/03/2011. Retrieved 04/23/2016.
- ↑ Matt Humbard and Joe Idoni. Milk The Funk Facebook group. 04/29/2017.
- ↑ Richard Preiss. Milk The Funk Facebook group thread on the frequency of Pediococcus caused ropiness. 08/20/2019.
- ↑ Ethanol tolerance of lactic acid bacteria, including relevance of the exopolysaccharide gene gtf. Pittet V, Morrow K, Ziola B. 2011.
- ↑ Exopolysaccharide production by Pediococcus damnosus 2.6 in a semidefined medium under different growth conditions. Maite Dueñas, Arantza Munduate, Aidé Perea, Ana Irastorza. 2003.
- ↑ Barley β-Glucans-Containing Food Enhances Probiotic Performances of Beneficial Bacteria. Mattia P. Arena, Graziano Caggianiello, Daniela Fiocco, Pasquale Russo, Michele Torelli, Giuseppe Spano, and Vittorio Capozzi. 2014.
- ↑ Beta-Glucans Improve Growth, Viability and Colonization of Probiotic Microorganisms. Pasquale Russo, Paloma López, Vittorio Capozzi, Pilar Fernández de Palencia, María Teresa Dueñas, Giuseppe Spano, and Daniela Fiocco. 2012.
- ↑ In Situ β-Glucan Fortification of Cereal-Based Matrices by Pediococcus parvulus. Adrián Pérez-Ramos, María Luz Mohedano, Paloma López, Giuseppe Spano, Daniela Fiocco, Pasquale Russo, and Vittorio Capozzi. 2017.
- ↑ Ethanol Tolerance of Lactic Acid Bacteria, Including Relevance of the Exopolysaccharide Gene Gtf. Vanessa Pittet and Kendra Morrow and Barry Ziola. 2011. DOI: https://doi.org/10.1094/ASBCJ-2011-0124-01.
- ↑ Coulon, Joana et al. “Lysozyme Resistance of the Ropy Strain Pediococcus Parvulus IOEB 8801 Is Correlated with Beta-Glucan Accumulation around the Cell.” International Journal of Food Microbiology 159.1 (2012): 25–29. Web.
- ↑ [https://pubmed.ncbi.nlm.nih.gov/19659698/ Ciezack G, Hazo L, Chambat G, Heyraud A, Lonvaud-Funel A, Dols-Lafargue M. Evidence for exopolysaccharide production by Oenococcus oeni strains isolated from non-ropy wines. J Appl Microbiol. 2010 Feb;108(2):499-509. doi: 10.1111/j.1365-2672.2009.04449.x. Epub 2009 Jun 30. PMID: 19659698.
- ↑ Zach Taggart. Milk the Funk Facebook group post on EPS from yeast. 01/16/2019.
- ↑ Exopolysaccharides from yeast: insight into optimal conditions for biosynthesis, chemical composition and functional properties - review. Iwona Gientka, Stanisław Błażejak, Stanisław Błażejak, Lidia Stasiak, Lidia Stasiak, Anna Chlebowska-Śmigiel, Anna Chlebowska-Śmigiel. 2015.
- ↑ Enhanced Bacteriocin Production by Pediococcus pentosaceus 147 in Co-Culture with Lactobacillus plantarum LE27 on Cheese Whey Broth. Carolina Gutiérrez-Cortés, Héctor Suarez, Gustavo Buitrago, Luis A. Nero and Svetoslav D. Todorov. 2018. DOI: 10.3389/fmicb.2018.02952.
- ↑ Antibacterial activity of Lactobacillus plantarum isolated from Tibetan yaks. Lei Wang, Hui Zhang, Mujeeb Ur Rehman, Khalid Mehmood, Xiong Jiang, Mujahid Iqbal, Xiaole Tong, Xing Gao, Jiakui Li. 2017.
- ↑ Growth optimization of Pediococcus damnosus NCFB 1832 and the influence of pH and nutrients on the production of pediocin PD-1. H.A. Nel1, R. Bauer, E.J. Vandamme and L.M.T. Dicks. Jan 2002.
- ↑ Purification, partial amino acid sequence and mode of action of pediocin PD-1, a bacteriocin produced by Pediococcus damnosus NCFB 1832. Bauer R, Chikindas ML, Dicks LM. May 2005.
- ↑ Tyramine Formation by Pediococcus spp. during Beer Fermentation. MARIA IZQUlERDO-PULIDO, JOSEP-MIQUEL CARCELLER-ROSA, ABEL MARINE-FONT, and M. CARMEN VIDAL-CAROU. 1996.
- ↑ Effect of tyrosin on tyramin formation during beer fermentation. Maria Izquierdo-Pulido, M. Carmen Vidal-Carou. 2000.
- ↑ Biogenic Amine Changes Related to Lactic Acid Bacteria During Brewing. MARIAI ZQUIERDO-PULIDO, JUDIT FONT-FABREGAS, JOSEP-MIQUEL CARCELLER-ROSA, ABEL MARINE-FONT, and CARMENVIDAL-CAROU. 1995.
- ↑ Isolation, Identification, and Characterisation of Beer-Spoilage Lactic Acid Bacteria from Microbrewed Beer from Victoria, Australia. Garry Menz, Christian Andrighetto, Angiolella Lombardi, Viviana Corich, Peter Aldred, Frank Vriesekoop. 2010.
- ↑ Jansen, Dave. Hors Category Blog. "Duivelsbier of Halle". 07/30/2016. Retrieved 01/31/2018.
- ↑ Microbial diversity and metabolite composition of Belgian red-brown acidic ales. Isabel Snauwaert, Sanne P. Roels, Filip Van Nieuwerburg, Anita Van Landschoot, Luc De Vuyst, Peter Vandamme. 2015.
- ↑ The Impact of Simple Phenolic Compounds on Beer Aroma and Flavor. Michael Lentz. 2018. doi: 10.3390/fermentation4010020.
- ↑ Justin Amaral. Milk The Funk Facebook comment on storing Pediococcus. 10/23/2017.